Figure 1: Schematic of Pilot Systems.
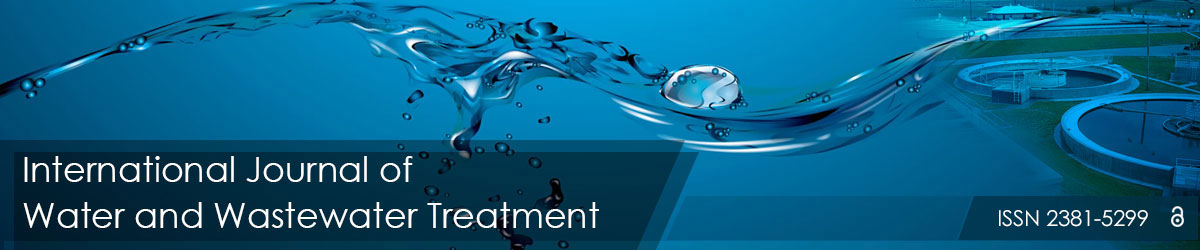
Full Text
Domènec Jolis* Albert Velasco
Wastewater Enterprise, Public Utilities Commission, Phelps Street, San Francisco, California, USA*Corresponding author: Domènec Jolis, Wastewater Enterprise, Public Utilities Commission, 750 Phelps Street, San Francisco, California, USA, E-mail: djolis@sfwater.org
Two grit removal systems were tested side-by-side for the treatment of wastewater from a combined-sewer system. The two units were operated at constant flows and variable grit loads ranging from 1.2 to 745 Kg/m3/h that covered the typical range of dry and wet weather conditions experienced at the treatment facility. The average mass grit removal for the multi-tray free vortex unit was 92.6% and it was 85.4% for the forced vortex system. Both units consistently failed to remove particles with sand equivalent sizes, which their respective overflow rates would otherwise predict. This observation can be explained by uneven force distributions over non-spherical grit particles with lumpy grease deposits and variable curvature that effect motion traverse to flow, increase buoyancy and cause particle lift.
Free vortex; Forced vortex; Grit separation; Multi-tray system; Particle buoyancy
Inorganic suspended particles with sizes between 0.05 and 1.0 mm constitute grit in wastewater. Inside treatment plants, grit increases the cost to operate and maintain unit processes through accumulation in tanks and conduits. In addition, grit can accelerate wear in moving parts of mechanical equipment such as pumps, heat exchangers and centrifuges. Therefore, grit removal systems are installed in the frontend of treatment plants with the objective of removing all grit that is practical and economical to do. Grit removal systems include three steps, namely, grit separation, where suspended grit particles are diverted from influent wastewater; grit washing, where organic matter both attached to grit particles or in suspension is removed from the grit slurry; and grit dewatering, where excess water is discarded to leave a clean, dry grit product that can be recycled or disposed, but only the first unit process is the focus of this paper. Grit can be removed by gravity since the density of a clean grit particle is larger than water, with a typical specific gravity of 2.65. However, interaction of grit with grease and other organic material in collection systems can significantly reduce the density differential between particle and water and cause real grit particles to settle at lower velocities than their size would predict using Newton’s Law of force balance [1].
For spherical particles, the settling (terminal) velocity [2] is obtained by equating the gravitational force to the frictional drag force as expressed in Newton’s law:
\[{v_p} = {\left( {\left( {\frac{{4g}}{{3{C_d}}}} \right)\left( {\frac{{{\rho _p} - \rho w}}{{{\rho _w}}}} \right){d_p}} \right)^{\frac{1}{2}}}\]
Where
vp is the settling velocity of the particle, in meter per second
g is the gravity acceleration, in meter per second squared
Cd is the drag coefficient, unit less
ρp is the density of the particle, in kilogram per cubic meter
ρw is the density of water, in kilogram per cubic meter
dp is the diameter of the particle, in meter
Also, real grit particles have odd shapes that deviate from round spheres and have one or two of their dimensions larger than the third, which further affects their effective settling velocities [3]. For this, it has been proposed to use the Sand Equivalent Size (SES) of grit particles for the design and assessment of grit removal systems. The SES of a grit particle is the size of a clean, round sand particle with a density of 2.65 g/cm3 that would settle at the same velocity. However, most systems are still designed based on particle size as generating SES is costly. A complete review of particle settling in turbulent flows applicable to grit removal from wastewater is available elsewhere [2,4].
San Francisco has a combined-sewer system with two watersheds draining into the Pacific Ocean and the San Francisco bay, respectively. The Southeast Treatment Plant (SEP) discharges into the bay and receives its raw sewage from three different sources, the main source being from the northeastern part of San Francisco and the other two being local sources in close vicinity of the treatment facility. The SEP is a high-purity oxygen activated sludge treatment plant designed for carbon removal only that includes pre-treatment (coarse and fine screening and grit removal), primary settling tanks and sodium hypochlorite disinfection. Typical effluent concentrations of Biological Oxygen Demand (BOD) and Total Suspended Solids (TSS) are less than 20 mg/L. Due to an aging aerated grit chamber with mechanical problems and an underperforming vortex separator, great amounts of grit passed through the existing infrastructure, mostly during rain events when the inflow to the plant increases by 400% to 11 m3/s, and caused severe operational problems and excessive maintenance costs. Thus, for 2014, the amount of grit that was not captured at the plant was on the order of 1000 metric tons.
Limited space and slow settling velocities of real grit particles have necessitated the adoption in urban wastewater treatment plants of compact grit removal technologies, with devices that use a vortex flow pattern being widely adopted. There are two types depending whether the vortex originates hydraulically or mechanically and depend on a tangential entry of the flow for circular motion and either shape (for the free vortex system) or an impeller (for the forced vortex system) to create velocity gradients between liquid and particle to ensure separation of the target particle size in the residence time of the unit, measured in minutes rather than hours typical of aerated grit chambers. The flow regimes of the two systems are different and affect how grit is collected after separating from wastewater, with the free vortex system discharging both streams near the center where the velocity gradient is maximum and the Forced Vortex System (FVS) discharging wastewater at the periphery where the velocity gradient is maximum in this case, and separated grit moving along the bottom of the device, under a separating plate and into a fluidized hopper. The main disadvantage of free vortex systems is their energy consumption, which is higher for smaller sizes of particles targeted for removal and has led to the development of the Multi-Tray Free Vortex (MTS) system that incorporates a variable number of stacked trays depending on the size of the grit particle to remove. MTS greatly increases surface area and minimizes settling distances, thus becoming very compact and energy efficient. Vortex systems for grit removal are proprietary and although their design must be based on Newton’s Law, their performance can only be confirmed after installation due to factors such as baffles; impeller shape and speed; flow entrance and exit; and distribution structures and devices that can affect particle removal according to manufacturers. Therefore, if the design criteria are not met during testing, it can lead to costly retrofits and higher operational and maintenance costs for the life of the infrastructure [5].
Section 1 introduces the work while Section 2 lists the objectives and Section 3 presents the materials and methods. Section 4 presents the results while Sections 5 and 6 respectively present the discussion and the conclusions.
The objective of the study was to side-by-side compare the performance of two wastewater grit removal technologies, namely a Multi-Tray Free Vortex System (MTS) and a Forced Vortex System (FVS), treating the same influent wastewater to better understand the physical mechanisms governing the removal of grit by each technology and how they affected removal efficiency. In addition, we assessed the dependence of grit removal on the characteristics and quantity of grit particles in wastewater.
There is very limited data about the particle size distribution and quantity of grit in combined sewer systems but a recent grit characterization effort at SEP provided the results in table 1. These data represent results from samples collected for two weeks and show great ranges in loading conditions throughout a single day, between the days of one week and between dry and wet weather. Also, significant changes in particle size distribution were observed, which in combination with the uncertainty in design parameters of proprietary technology as described above, justified the need for parallel testing of pilot systems capable to remove 95% of particles greater than 106 μm or better, under a wide range of loads that were representative of conditions at the SEP.
Dry Weather | Wet Weather | |||
Influent Grit Load (g/m3) |
Average | Range | Average | Range |
Daily | 30 | 18-45 | 144 | 36-372 |
Hourly | 1.2-84 | 6-745 | ||
Particle Size (µm) | Percent | Percent | ||
d>300 | 28 | 40 | ||
300>d>150 | 50 | 43 | ||
150>d>106 | 18 | 14 | ||
d<106 | 4 | 3 |
Table 1: SEP Grit Characteristics.
Ratios of maximum day to average day load and the range of average loading conditions in table 1 are typical of values published in the literature [1] for combined-sewer systems and therefore data gained from this work should be representative of these systems. On the other hand, only dry weather data may be applicable to separatesewer systems based on typical ratios and average loads [6].
This study was carried out at the SEP in San Francisco, CA and used one MTS pilot unit with rated capacity for 0.23m3/s and one FVS pilot system with rated capacity for 0.31m3/s. The MTS was designed to remove 95% of incoming particles equal or larger than 75 μm in diameter whereas the FVS design targeted 95% of incoming particles with a diameter equal or larger than 106 μm. The pilot units received flow from a side stream downstream of the bar screens of the treatment plant. The screened wastewater was pumped with constant flows of 0.23 and 0.27 m3/s to the MTS and FVS pilot units due to limitations in pumping capacity. Figure 1 shows a schematic of the pilot systems. The pilot units were operated during the dry weather season for nine weeks and for four weeks during the wet weather season to capture the full range of grit loading conditions observed at the SEP.
Two-inch grit sampling taps were installed in the influent and effluent lines of both pilot units and plumbed to grit settlers. Grit settlers were constructed from 208-liter plastic complete-drain inductor tanks with an influent port and a discharge weir. Flow entered the tank and was diverted to the side with a 90° elbow to reduce the velocity and turbulence. Grit settled to the bottom of the tank and wastewater exited through the top weir. The surface area of the settler in figure 2 is 0.29m2 and the flow through it was 0.5 l/s for an overflow rate of 1.74 × 10-3 m/s and slower than the settling velocity of a 50 µm sand particle of 2.12 × 10-3 m/s, thus ensuring the capture of virtually all grit particles. At the end of the sampling period, the sample was decanted from the bottom of the settler and split into aliquots to facilitate the rinsing of organic material. The aliquots were then combined into a single composite sample and immediately classified through four sieves in five different diameter ranges as seen in table 2. The weight of each fraction of grit particles was measured using methods 2540B and 2540E of Standard Methods (APHA-AWWA-WEF, 1998) [7].
Figure 2: Grit sampler.
diameter<106 µm |
106 µm<diameter<150 µm |
150 µm<diameter<212 µm |
212 µm<diameter<300 µm |
d>300 µm |
Table 2: Grit particle sizes.
For the duration of the study, the mass of grit entering and leaving the two pilot units was measured and this data is presented in figures 3 and 4, which address the performance of the MTS and FVS and their dependence on grit load. Figure 3 shows to what extent each system removed incoming grit over the full range of loading conditions observed during the study. Grit loading appears in the x-axis and is normalized by the average load of 30 g/m3 (250 pounds (lbs)/106 gallon (Mgal)) experienced throughout the three months of operating the pilot units. Normalization of loading conditions is later used to analyze the performance of the MTS and FVS for the different grit particle sizes in table 2.
Figure 3: Multiple Tray vs Forced Vortex Systems Performance.
Figure 4: Grit Pass Through vs Grit Load.
As can be seen in figure 3, the MTS performed better than the FVS for all loading conditions. High grit loading conditions at the SEP typically occur during wet weather events when surface runoff enters the combined sewer system and the water velocity increases, causing more and larger grit particles to arrive at the treatment plant. In those conditions (normalized grit loading of 2.0 or greater) the MTS clearly outperformed the FVS. At normalized grit loading of 0.5 or less, the FVS performed very poorly, in particular at very light loading conditions (i.e., 7.5 g/m3 or less) when grit passing through the system exceeded 30% of the incoming load. Thus, only for average loading conditions, the performance of the two pilot systems was comparable albeit the MTS still performed somewhat better than the FVS.
Figure 4 also clearly demonstrates that the MTS performed better than the FVS under the conditions tested. The linear correlations between grit loading and grit pass through included in figure 4 are statistically significant at the 95% confidence level (T-test of the differences) and show that on average the MTS removed 92.4% of the incoming grit load whereas the FVS removed 85.4%. In other words, the FVS let through grit at twice the rate than the MTS did.
The regression factor of a linear correlation provides an estimate of how well the free variable predicts the value of the dependent variable. In this case, the observed regression factors for MTS and FVS suggest that the performance of grit removal systems is mostly dependent on the loading conditions, with between 71 and 76% of the grit passing through being predicted by grit load. Moreover, the scatter in the data in figure 4 can be attributed to grit characteristics such as size, shape, and density of the grit particles, in combination with hydraulic effects in the separation devices, and account for about 25% of the observed response of the pilot units.
On different days throughout the program, influent and effluent grit samples were collected and sieved as described in the Methods sections to gain information on the response of the grit separation systems to grit particles of various sizes. Table 3 presents the aggregated data in three ranges of normalized loading values, representing light, medium and heavy loading conditions. For all particle sizes and loading conditions, MTS removed more grit mass than FVS clearly demonstrating that it was the better technology for the conditions tested. Both systems struggled with light loading conditions, but showed a very consistent performance over all other loading regimes.
MTS | FVS | |||||
Normalized Loading | Normalized Loading | |||||
Grit Size | NL<0.4 | 0.4>NL>1.6 | 2.0>NL>4.0 | NL<0.4 | 0.4>NL>1.6 | 2.0>NL>4.0 |
diameter<106 μm | 56.6 | 67.3 | 70.1 | 54.1 | 64.9 | 63.3 |
106<diameter<150 μm | 70.9 | 82.3 | 82.6 | 55.0 | 62.8 | 61.5 |
150<diameter<212 μm | 78.8 | 89.2 | 89.4 | 57.9 | 65.9 | 67.0 |
212<diameter<300 μm | 89.0 | 95.2 | 95.8 | 82.5 | 91.4 | 90.9 |
diameter>300 μm | 91.1 | 97.8 | 98.3 | 84.3 | 95.8 | 95.3 |
Overall | 86.7 | 93.3 | 94.6 | 78.3 | 88.1 | 87.6 |
Table 3: Percent Removal by Weight of Grit of Different Sizes by MTS and FVS and Overall.
Larger grit particles were removed more effectively by both systems, reflecting their respective design criteria, even though they were clearly not met for particle sizes up to 212 μm. The FVS did particularly poorly for particle sizes ranging from 106 to 212 μm across all loading regimes when compared to the MTS, which contributed to its overall worse performance. Also of note is the fact that both units appeared to remove large particles (i.e., diameter larger than 212 μm) less efficiently under light loads, although the two systems are designed to completely remove them.
The Overflow Rate (OFR) of a settler has the dimensions of velocity and can be used to analyze the particle size that will be removed in the tank by equating it to the particle’s settling velocity using Newton’s Law, thus providing the basis for the design of grit separators [8,9]. In this study, the overflow rates were 0.078 m/s for the MTS and 0.366 m/s for the FVS, which translate to the settling velocities of particles with a SES of 95 and 206 μm, respectively. When compared with the design criteria of these systems, the MTS appears to effectively be designed based on OFR albeit with a slightly optimistic expected performance when compared to results from this study that the overall mass removal under all load conditions tested was slightly over 92%. Moreover, performance data shows a contradictory picture: a design criterion of 95% removal of particles 75 µm but observed removal rates for particle sizes below 212 μm consistently below expectations [10]. On the other hand, there is an evident discrepancy between design criteria and OFR for the FVS and the overall observed mass removal of around 85% would suggest neither OFR nor design criteria accurately predict actual performance, even after considering that the contribution of particles smaller than 106 μm to the total mass load was between 3 and 4% (Table 2). Moreover, removal rates of particle sizes between 212 and 300 μm did not conform to the unit’s OFR either. Thus, following the design criteria of manufacturers to design a grit separation device will lead to more grit passing through into downstream unit processes than anticipated, and pilot testing in combination with grit characterization would seem advisable to gain as much insight as possible before such a system is implemented at treatment facilities. However, the less costly combination of a grit characterization study and proper OFR selection may suffice to install a well-performing MTS grit separation device for combined-sewer systems.
Figure 3 clearly demonstrates MTS to be more effective at removing grit than FVS under the test conditions as would be expected from the respective operating OFR with more than 7% more mass passing through the FVS overall, which for the 2014 SEP data reported above translates to about 150 tons/year of excess grit affecting downstream processes. This result agrees with the units respective operating OFR and observed improved grit removal in FVS when influent flow rates are lowered [5].
Grit quality, namely particle shape and degree of coverage with grease and other organic matter play an important role in grit removal by affecting settling characteristics [3]. However, according to figure 4 between 24 and 29 % of observed removal rates cannot be explained by grit loading alone and the hydraulic regimes in MTS and FVS appear to hinder the separation of grit from wastewater [11,12].
The viscosity of wastewater causes the flow around grit particles to slow down while being in contact with the surface, forming a boundary layer [13]. Due to the variations in curvature in real particles, the boundary layer will separate from the body forming vortices that change the pressure distribution along the surface of the particle. When vortices are formed asymmetrically around the body (with respect to its mid-plane) due to shape or uneven grease coverage, different lift forces develop on each side of the particle that cause motion traverse perpendicular to the flow line [13], increasing buoyancy and hindering removal. Moreover, separated grit moves along the bottom of the FVS to reach the hopper in the center of the unit and, if vortex-induced vibrations lift a particle off the surface, the upward flow near the discharge structure of the FVS can carry it over with the treated effluent [14]. Similarly, grit traps are often installed in combined sewer systems to remove grit before the material reaches the treatment facility but grit re-entrapment into the bulk of the liquid can occur if not designed properly [15].
These mechanisms may help explain the results in figure 4 as well as the lack of performance of MTS and FVS when compared to their respective operating OFR. In MTS this is apparent for particle sizes between 106 and 212 μm, with larger, heavier size particles being removed at expected rates as vortex-induced lift forces have less influence [16]. In FVS, decreased removal extends to particle sizes between 212 and 300 μm as wastewater velocities are greater due to its higher OFR. Light loading conditions seem to exacerbate the problem in part because the quantity of large particles is smaller and perhaps because there are lesser opportunities for particles to coalesce into larger ones that are more readily removed.
The requirement to remove 95% of particles greater than 106 μm may not be necessary to adequately protect downstream unit processes from the effects of grit for treatment facilities in combined-sewer systems like the SEP. In this study, for loading conditions between 12 and 120 g/m3 (100-1000 lbs/Mgal), the average mass grit removal was 94% while the specific removal rates for particle sizes smaller than 212 μm was less than 90%. The greater weight and concentration of large particles in such systems is helpful with this metric. Stricter design criteria may however be necessary for separate-sewer systems, unless loads are small (e.g., 7.5 g/m3) and so is the quantity of grit passthrough.
Grit separation in MTS was more efficient than in FVS because of their different operating OFR and hydraulic regimes. On average the MTS removed 92.4% of the incoming grit load whereas the FVS removed 85.4%. Neither system performed as would be expected from their OFR and grit removal rates were particularly poor under light loading conditions. Non-spherical shape and uneven grease coverage of real grit particles account for the scatter observed in mass grit removal data. Vortex-induced vibrations on non-spherical, buoyant particles could cause grit that should settle under operating OFR to avoid capture decreasing the effectiveness of tested systems for grit separation. However, MTS would still sufficiently protect downstream unit processes in combined-sewer treatment facilities by removing 94% of grit by mass over most observed loading conditions. Grit characterization and in-situ testing of units are greatly recommended before design of grit removal systems.
- Metcalf and Eddy (2014) Wastewater Treatment and Reuse. 5th edition, McGraw Hill, New York.
- McGauhey PH (1956) Theory of sedimentation. J AWWA. [Ref.]
- Sober J, Gwinn A, Ortiz C, Correa R, Sherony M (2018) Grit settling velocity: A visual comparison of clean sand and wastewater grit. Water Environ Fed 2018: 2121-2127. [Ref.]
- Schlichting H (1979) Boundary layer theory. McGraw-Hill, New York. [Ref.]
- Chien MH, Borys A, Wong JL (2010) Computational Fluid Dynamics Analysis of a Vortex Grit Removal System. Water Environ Fed 2010: 6020-6032. [Ref.]
- Krishnappan BG, Exall K, Marsalek J, Rochfort Q, Kydd S, et al. (2012) Variability of settling characteristics of solids in dry and wet weather flows in combined sewers: Implications for CSO treatment. Water Air Soil Pollut 223: 3021-3032. [Ref.]
- Walter WG (1961) Standard Methods for the Examination of Water and Wastewater. 11th edition, American Journal of Public Health 51: 940. [Ref.]
- Meroney RN, Sheker RE (2015) Removing grit during wastewater treatment: CFD analysis of HDVS performance. Water Environ Res. [Ref.]
- Plana Q, Lessard P, Vanrolleghem PA (2020) Dynamic grit chamber modelling: dealing with particle settling velocity distributions. Water Sci Technol 81: 1682-1699. [Ref.]
- Lee JH, Bang KW, Choi CS, Lim HS (2010) CFD modelling of flow field and particle tracking in a hydrodynamic stormwater separator. Water Sci Technol 62: 2381-2388. [Ref.]
- McNamara BF, Kochaba T, Griffiths J, Brook D (2008) Grit vs grit. A pilot evaluation comparing two grit removal technologies. Water Environ Fed 2009: 784-793. [Ref.]
- Luyckx G, Berlamont J (2004) Removal efficiency of swirl/vortex separators. Urban Water J. [Ref.]
- Naudasher E, Rockwell D (1994) Flow-induced Vibrations: an Engineering Guide. Taylor & Francis. [Ref.]
- Plana Q, Pauléat A, Gadbois A, Lessard P, Vanrolleghem PA (2020) Characterizing the settleability of grit particles. Water Environ Res 92: 731-739. [Ref.]
- Faram MG, Harwood R (2003) A method for the numerical assessment of sediment interceptors. Water Sci Technol 47: 167- 174. [Ref.]
- Samstag RW, Ducoste JJ, Griborio A, Nopens I, Batstone DJ, et al. (2016) CFD for wastewater treatment: an overview. Water Sci Technol 74: 549-563. [Ref.]
Download Provisional PDF Here
Article Type: RESEARCH ARTICLE
Citation: Jolis D, Velasco A (2022) Side-by-Side Testing of Grit Separation Units. Int J Water Wastewater Treat 8(2): dx.doi.org/10.16966/2381- 5299.185
Copyright: © 2022 Jolis D, et al. This is an open-access article distributed under the terms of the Creative Commons Attribution License, which permits unrestricted use, distribution, and reproduction in any medium, provided the original author and source are credited.
Publication history:
SCI FORSCHEN JOURNALS
All Sci Forschen Journals are Open Access