Figure 1: Uranium exposure based on different kinds of bedrock adopted from [4].
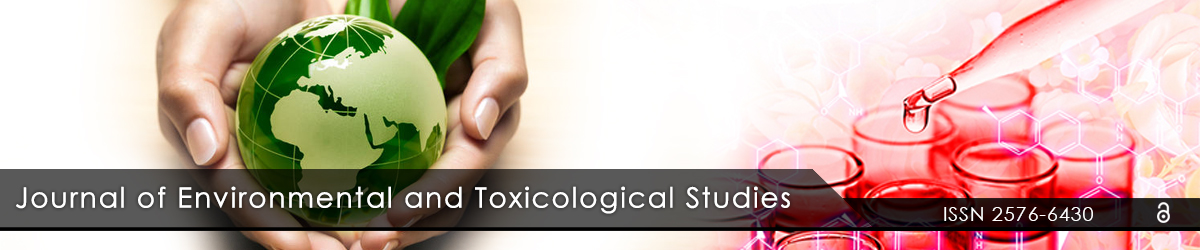
Full Text
Meaad Al Jassim1 Rima Isaifan1,2*
1Sustainable Development Division (SDD), College of Science and Engineering (CSE), Hamad Bin Khalifa University (HBKU), Qatar Foundation (QF), Education City, Doha, Qatar2Qatar Environment and Energy Research Institute (QEERI), Hamad Bin Khalifa University (HBKU), Qatar Foundation, Doha, Qatar
*Corresponding author: Rima Isaifan, Qatar Environment and Energy Research Institute (QEERI), Hamad Bin Khalifa University (HBKU), Qatar Foundation, Doha, Qatar; E-mail: risaifan@hbku.edu.qa
Radon pollution is one of the critical challenges that we face today in the field of pollution. It is considered as a radioactive component emitting continuous radiation throughout its short life span. Recently, many researchers showed interest in studying the effects of radon on human health since it is known as one of the most common indoor air-contaminants due to its carcinogenic properties. It can cause lung cancer and it has been estimated that around 3,000 deaths take place every year in Canada due to the exposure to radon. Moreover, several works have discussed the domestic exposure to radon and its effects on childhood cancer. On the other hand, literature has demonstrated that the main impacts of radon on the environment were related to air and water contamination. In fact, it has been identified that there are different sources of indoor radon that include building materials, tap water, and geological substratum. Several methods for radon transportation had been identified and the highest concentration of radon is in building materials which is as a result of the high porosity of isotropic sand. In this paper, around 70 studies were screened to have a better understanding of indoor radon sources, methods of radon indoor concentration measurements and prediction, its effects on human health and the environment in addition to the techniques used to mitigate or reduce its impact on human’s health and human welfare.
radon; Indoor air pollution; Health risks; Air quality; Water contamination
Radon (Rn) is obtained in the air as gas phase. It is a radioactive component that eventuates naturally and emanates from the decomposition of uranium-238 and thorium, all present in the earth’s crust [1,2]. Radon’s isotopes 222Rn and 220Rn are the most common and are deemed as indoor radon nuclide with a half-life of 222Rn of about ~3.8 days [3,4]. 222Rn and 220Rn decay first polonium 218 and 215 respectively. Then they decay into lead through the emission of α particles. These particles provoke an intense, continuous, natural radiation as a result of their short life span.
222Rn decomposes by alpha particle emission into charged progeny, which attaches to air or water particles, just like all radon isotopes. Radon isotopes can cause DNA damages in sensitive cells since they are readily inhaled. This is because of their ability to penetrate deep into the respiratory tract. Its exposure was initially associated with lung cancer incidence in underground miners [4-7]. Additionally, this has caused a growing interest in the study of radon’s effect on human health since it has been globally identified as a common indoor-air contaminant with carcinogenic properties. This has led to measuring radon concentration indoor in many countries around the world (specifically in buildings) to accomplish policies and regulation requisites [8-10]. Given that the robust relationship between underlying geological formations and building structures enhances the level of indoor radon, its concentration inside buildings has been studied in line with the radon gas from soil and construction materials [11].
This paper involves conducting a review of indoor radon sources and effects by reviewing around 70 articles in this topic. First, it provides an overview of radon sources and transportation mechanism. In addition, it discusses the geographic and climate factors of radon distribution. Then, it focuses on evaluating radon risk and human exposure in addition to its environmental impact. We then present appropriate mitigation measures for this issue. To this end, several gaps in this topic are highlighted as possible research interest areas and then the study is closed by several concluding remarks.
Sources of radon
There are three main sources of indoor radon: the geological substratum, building materials used in dwelling, and tap water. Therefore, it is evident that contamination may occur either through the soil-air interface or directly at the water interface.
Soil: The main contributor of radon is the soil, and up to 10 percent of it is emancipated in the atmosphere, where it may travel for long distances [12]. Hazards affiliated with radon submission are assessed by radiometric surveys in the mapping of areas of high radon concentrations [13,14]. The radon gas originates from soils and amasses in small building areas. Measurements take into account radon gas concentration itself as well as soil permeability. The European Commission in 1990 recommended the reference levels of 400 Bq/m3 and of 200 Bq/m3 for prevailing and subsequent buildings respectively [4].
222Rn levels inside a structure closely correspond with the Uranium content of the latent strata. Vital contributors to the radon content of indoor-air are bedrocks such as granite, clay and carbonated rocks [4]. Figure 1 showed the Uranium exposure based on different kinds of bedrock.
Additionally, karstic limestone formations can also serve as a means of radon travel into structures, irrespective of the fact that they are highly fractured [7]. Therefore, the ground is the significant source of radon in buildings. Basements are more exposed to radon elements due to their limited ventilation and closer contact with the ground as collated to other parts of the building [15]. Several researchers have confirmed that there is a strong relationship between uranium concentration in the soil and the level of indoor radon concentration [11,16,17].
Construction materials: Another crucial source of indoor radon is the building materials. This happens particularly when granite rocks are utilized, as in the case of Galicia in Spain, which has the highest mean radon concentrations measured at 118 Bq/m3 , which is double the average concentration in Spain as a whole, due to its geologically fractured soil [4,14]. According to Denman et al. [18] a study done in the UK in 2007 exhibited that the content of radon in building materials leads to a heightened level of radon in many houses. Additionally, it is eminent from a further study that pores and cracks in concrete blocks may also amplify exposure to radon [12,19]. Figure 2 is shows the main entrance routs for radon at home where A is pointing to a crash in concrete slabs; B is showing empty point behind block veneer walls that stand on hollow; C is showing openings in concrete blocks; D is showing floor and wall junctions; E is soil; F is drain from open sump; G, mortar joints; J, building materials, such as some rock; K, water (from some wells).
Figure 2: Main entrance points for radon in home taken from [12].
Other Sources: Diverse studies to examine the sources of radon exposure have been conducted across the globe. As a matter of fact, the existence of radon in tap water raised the levels of indoor radon vulnerability by 100 Bq/m3 [4]. The data collected recently have been contrasted to show that spas and thermal baths have become the major sources of waterborne exposure [12]. However, water and spas are not the only predominant sources of exposure. Depending on the lithology and altitude of structures, indoor radon levels also differ. Kropat et al. [20] has performed a study that manifested that carbonate rocks in Alps emits less radon as compared to carbonate rocks in Jura. The study substantiates the concept that there exists a linear correlation between indoor radon concentration and outer altitude.
Radon transport mechanism
Porous and isotropic sand play important roles in radon transportation to building material. First, diffusive transport where the movement of radon will be based on differences in radon concentration is considered as main processes to be exposed to radon atoms that are available in sand over the walls in houses. Secondly, the movement of radon atoms by wind blooms which is called advective transport. Advective transport mechanism allows radon to enter the building through porous media for example, porous concrete made from sand. In addition, it plays an important role to transport radon atoms from soil further to buildings and living areas [21]. Figure 3 demonstrates radon transport mechanism the ∆C= change in radon concentration and ∆P= change in pressure.
Figure 3: Radon transport mechanism (diffusion and advection) [21].
The diffusive movement of radon atoms can be described by Fick’s law equation of diffusion
j =− Dm. ∇Ca (1)
Where j is flux (Bqm-2·s-1), ∇Ca is change in radon concentration (Bqm-4) and Dm is the coefficient for the molecular diffusion (m2 ·s-1) [21].
Geological and climatological aspects
The World Health Organization (WHO) has developed a survey to measures the concentration of radon around the world based on the data collected from The United Nation Scientific Committee on the effect of atomic radiation 2000 (UNSCEAR 2000) and literatures. Figure 4 showed the radon concentration around the world [22]. The map showed that the level of radon in The Kingdom of Saudi Arabia is around 7-50 Bq/m3, while it increases in Europe and America to the range of 20-104 Bq/m3.
Figure 4: Radon level by country taken from [22].
There is a strong relation between geological and climatological features with radon level in air. A study conducted in Brazil (2003) showed that there are some differences in radon concentration in seasonal and daily prospective. The study showed that there is an inverse correlation between outdoor air temperature and radon level in air, in which radon level is higher in the early morning with gradual decrease till the noon. In addition, the study recorded higher level in winter comparing to summer linked with an inverse correlation with rainfall [23]. The results were in line with the study conducted by Gopala krishnan et al. [24] in India whose study showed higher level of radon in winter.
In general, several studies conducted in this field proved the association between radon concentrations and geological factors. The concentration of indoor radon in Cambrian to Lower Devonian found to be high and medium due to bedrock type soil while in Jurassic showed medium level [25]. Figure 5 showed the concentration of radon in Belgium.
Figure 5: The concentration of radon in Belgium [25].
Impacts of radon pollution
Radon health impact: Besides smoking, the World Health Organization reported that radon is the leading lung cancer cause accounting for up to 14 percent of cases globally [8]. Every year, Canada associates about 3,000 deaths with exposure to radon [26]. The United States Environmental Protection Agency (2016) estimates that about 21,000 people die from lung cancer associated with exposure to radon [27]. In the United Kingdom, a study by Gray et al. [28] showed that approximately 1,100 deaths are linked to exposure to radon. Exposure to radon even in small quantities could cause lung cancer. However, there exists no evidence providing the minimum exposure level that is acceptable without posing risk [29]. Altogether, numerous smokers who might not have contracted lung cancer might suffer from lung cancer due to exposure to radon. This is because cigarette smoking and lung cancer have been found to have a synergistic relationship as a lung cancer cause [30].
Humans suffer exposure from radon through four principal methods. These include ingestion, inhalation, injection, or absorption [31]. Upon groundwater percolating via uranium-rich rocks, it contains huge radon gas levels, which the public then ingests through drinking water. In addition to ingestion, human beings are exposed to radon through inhalation. Thus, groundwater presents with two exposure pathways. These include inhaling air containing radon that groundwater releases and drinking water [31]. However, rock and topsoil beneath the ground are considered the main sources of human exposure to radon-progeny in homes [14]. Akbari et al. [32] explain that population exposure to radon normally happens indoors. This is particularly common in small dwelling spaces although the exposure level differs by location. This variation in levels depends on building construction materials, ventilation, geological elements, and the atmospheric conditions indoors. Radon gas levels can accumulate indoors particularly at the lower building levels.
The spontaneous decay of radon atoms into radioactive atoms causes the release of radioactive atoms. These atoms are popularly known as radon progeny [33]. Upon inhalation, radon’s radioactive decay products could stick to the cells that line up in the lungs. This exposes the bronchial epithelial cells, which are quite sensitive, to alpha radiation. This exposure has the potential to trigger lung cancer [34]. On the other hand, inhaling high levels of radon can trigger mutation of the DNA (Deoxyribonucleic Acid) being a radioactivity source. This increases cancer risk through deposition of decay products within the epithelium of the lungs [35].
It is noteworthy that inhalation of high concentration levels of radon for long periods is what poses a greater risk to lung cancer [36]. Radon is nearly exhaled completely after being inhaled because it has a long half-life of about 3.82 days and is an inert gas. On the other hand, its progenies and especially its electrically charged daughters, 214Po and 218Po have short half-life can attach to particles of smoke or dust in indoor air. The inhalation of these particles causes their movement to the lungs. It is upon decay that they irradiate the tissue damaging cells to increase the risk of getting lung cancer [8]. The lung cancer fraction associated with radon indoor pollution ranges from 3 to 14 percent. This percentage depends on the average concentration of radon within the concerned country and the methods of calculation [8].
Globally, numerous epidemiological research studies estimating the relationship between lung cancer and in-house exposure to radon in general populations have been completed. Nonetheless, the evidence about the link between residential exposure to radon and the risk of getting lung cancer is not conclusive [36]. A fair summary of these studies has been presented in the three meta-analyses completed by Zhang et al. [37], Pavia et al. [38], and Lubin et al. [39] in three different decades. In all these three meta-analyses, a significant positive relationship between lung cancer risk and radon indoor. More recently, the meta-analysis by Garzillo et al. [36] focused on case-control studies only to ensure larger global coverage. The findings showed evidence of strong correlation between residential radon exposure and increased lung cancer risk.
Domestic radon exposure and risk of childhood cancer
Several studies have been conducted concerning domestic radon exposure and the risk of childhood cancer. However, the findings of such studies have not been consistent. For example, Raasschou Nielsen et al. [40] conducted a case-control study covering 922 cases of cancer in the Central Nervous System (CNS) and 1,153 leukemia to investigate the role of domestic radon in childhood cancer in Denmark. Their findings demonstrated that exposure to indoor domestic radon had an association with increased risk of lymphatic leukemia. However, there were no other significant correlations reported between exposure to indoor domestic radon and other childhood cancers. This study by Raasschou Nielsen et al. [40] was the only one found reporting an association between childhood cancer and domestic radon exposure over the past 10 years.
Most of the other studies have reported no evidence of an association between childhood cancer and domestic radon exposure. For example, Hauri et al. [41] conducted the first known cohort study for 1,332,944 children below 16 years living in Switzerland and based on the census data of 2000. Their study period had 258 CNS cancer and 283 leukemia cases. Using a nationwide model for predicting radon developed from 35,706 measurements of radon, they estimated radon exposure based on the home address of the children. Although the study lacked the children’s residential history for those born before 2005, the average concentration of radon was 77.7 Bq/m3 (Becquerels per cubic meter). In the end, indoor domestic exposure to radon was not found to be related to childhood cancer risk.
In their case control research, Kendall et al. [42] also investigated the role of radon exposure on childhood cancer with focus on leukemia in the Great Britain. Their study population comprised 36,793 cases of cancer, among which CNS tumors and lymphoid leukemia were 7,267 and 6,585 respectively. Estimates of radon exposure were based on the residence of the mother at childbirth and computed based on a predictive model comprising 460,000 dwellings. Upon grouping the radon findings based on geological boundaries and subsequent 1 km grid squares, they reported a 21.3 Bq/m3 concentration of radon. However, the findings showed that neither childhood cancer nor childhood leukemia were associated with domestic indoor exposures to radon.
Peckham et al. [43] investigated the relationship between childhood cancer risk and exposure to residential radon. They used childhood lymphoma incident in Texas based on 2,147 cases obtained from the Texas Cancer Registry for the years 1995 through 2011. The researchers sourced the regional average of radon concentrations from the Texas Indoor radon Survey and connected this to area of residence during diagnosis with cancer. Their finding showed no relationship between residential exposure to radon and Burkitt lymphoma, Hodgkin lymphoma, and lymphoma in general. The conclusion was consistent with that of other studies indicating inadequate evidence for the existence of a negative or positive association between childhood cancer and domestic exposure to radon.
In brief, two cohort-based studies (37 and 39) and the case-control study by Kendall et al. [42] did not find evidence to indicate that domestic exposure to radon increases the risk of cancer in children. The only study that found a correlation between domestic exposures to radon was the case-control study performed about a decade ago in Denmark by Raasschou-Nielsen et al. [40].
From the review in this section, it appears that the most common approach is the use of individual data and radon prediction models. This is possibly so because the incorporation of radon prediction models is likely to enable the inclusion of a bigger children population thereby reducing the occurrence of selection bias. However, it is noteworthy that different studies relied on different methods of determining residence. These included residences of the mother at childbirth (38), residence of the child at the time of diagnosis (38), and general residence data [41].
Radon’s environmental impact
The most immediate environmental impact of radon is the contamination or pollution of water and air quality [44]. Areas that have higher risk of indoor radon have shown an association with locations that are known to have high volumes and levels of radon activity in the soil underground. Radon in the underground soil affects the indoor activity level of volumetric radon at a large scale. There is need to acknowledge that radon being an inert gas is subject to influence by numerous factors that could change as it moves from its original place due to the activity of volumetric radon [45]. Some of the most important factors that affect the rate of radon infiltration and effect on the environment include soil permeability, moistures, and porosity, the diffusion coefficient of radon, rate of airflow in gaps, the area of soil contact, change in rate of air, room volume, and pressure variation between the ground surface and air trapped in soil pores [44].
The existence of radon, and especially its most stable isotope, 222Rn, in water sources such as rivers, underground water, raindrops, springs, and lakes poses the risk of water contamination as an environmental impact [46]. The 222Rn density varies in different underground water conditions. This mainly depends on numerous factors including meteorology, hydrology, geology, amount of parent radionuclides, and the nuclides absorbed by soil and rocks [47]. Geological factors like faults (structural parameter) and lithology are among the most crucial variable factors that determine the solubility of radon in water [48,49]. This explains the rationale for ranking the existence of faults close to water resources as critical in determining the concentration levels of radon and the potential environmental impact [50].
Various studies have reported different environmental impacts of radon on soil and water in different countries and regions. For example, Al-Nafiey et al. [51] reported a mean of between 0.21 Bq/l and 0.297 Bq/l in water for irrigation in Cameron Highland although this was within the acceptable limits of domestic and agricultural use. M. Shakir Aswood et al. [52] investigated the concentration of radon in a soil sample obtained from Cameron Highland and reported a high concentration level of 198.44 Bq/m3. Aswood et al. [53] reported the highest radon concentration levels in phosphorous samples (634.01 Bq/l) and the lowest in cow dung manure at 79.25 Bq/l. Saidi et al. [54] reported a highest radon concentration average of 14.7 Bq/l in well water for irrigation and drinking while tap water had a mean of 5.37 Bq/l radon concentration.
Measurement of radon indoor concentration
Since it was shown that radon indoor concentration would expose human health to different levels of risks, it is of critical importance that its concentration shall be measured. Several works have demonstrated theoretical and experimental methods for radon indoor concentration in some countries around the world. One of the interesting groups of research in this field is reported by Singh et al. [55,56], S. Komal et al. [57], BS Bajwa et al. [58]. In their recent work, Singh et al. [56] have reported on the calculation of indoor radon concentration calculations in selected South-East Haryana, India. Those dwellings were mainly made of mud and wood as building material. The authors used a three dimensional semi-empirical model to predict indoor radon concentration with theoretical calculations. This theoretical work was validated with experimental measurements over three different building materials to analyze radon exhalation diffusion length along with physical dimensions of observation area to calculate indoor radon concentration. The three building materials investigated were mud, brick and cement from which houses are constructed locally in South-East region of Haryana.
The experimental work to carry out radon exhalation from the building materials was conducted via an active measurement technique. This method involves the utilization of a scintillation radon monitor (SRM) as shown in figure 6 [56].
Figure 6: radon exhalation mass experimental set-up using SRM (Scintillation radon Monitor) [56].
The results showed that radon concentrations obtained via the prediction model have slightly varied from the experimental measurements due to different actual ventilation rates in the dwellings included in the study. Despite this slight variation, all models showed good linear correlation with the measured concentration values. Some of the experimental data obtained is shown in table 1.
Building material | Measured 222Rn exhalation rate |
Density (Kg· m-3) | Calculated radon diffusion length (m) |
Brick powder | 12.31 ± 1.14 mBq·Kg-1·h-1 | 1623 | 1.22 |
Concrete | 20.47 ± 0.2 mBq·m-2·h-1 | 2300 | 0.41 |
Brick fired | 0.8 ± 0.02 mBq· m-2·h-1 | 1800 | 0.09 |
Cement Portland | 3.73 ± 0.50 mBq·Kg-1·h-1 | 1506 | 1.61 |
Table 1: Measured exhalation rates, density and radon diffusion length of various building materials used in study [56].
In a follow up study, Singh et al. [55] attempted to update the old radon data available for Hamirpur district in India by measuring the concentration of indoor radon, thoron and their decay products using the newly developed radon /thoron discriminating diffusion chamber with single entry face (Figure 7), direct radon and thoron progeny sensors (DRPS/DTPS), respectively [58].
Figure 7: Schematic diagram of pinhole-based twin cup radon / thoron discriminating dosimeter [58].
The measurements have been carried out in 75 dwellings of 14 different villages and the results showed that high values of earlier reported radon concentrations were mainly due to thoron interference in the Solid State Nuclear Track Detector. Nevertheless, to correlate the measured average concentrations with human health impact, it was found that the measured values are within the reference levels reported for the annual inhalation dose recommended by International Commission on Radiological Protection (ICRP).
The results of the average radon concentrations obtained by Singh et al. [58] were almost 8.5 times less than the values reported earlier using the bare Solid State Nuclear Track Detector method (SSNTD) technique. This variation might be due to the interference of thoron and its decay products in the environment which gave an over estimated values of radon concentration in the vicinity of the experimental study.
Various authors (Lichtenstein et al. [59], WHO [8] and Larsson et al. [60]) have discussed different approaches for reducing radon and mitigating its effects. The review of existing literature led to the identification of several approaches for reducing radon exposure. However, the WHO (2009) Handbook contains a summary of six protection measures against radon. These include active and passive depressurization of the soil, surface sealing, membranes and barriers, and ventilating occupied and unoccupied spaces. After analyzing various measures, the most effective standalone measure for preventing radon within airtight constructions is the installation of active subslab systems of depressurization.
On the other hand, some researchers [61] have shown that the failure rate of radon -proof membranes is worryingly high. This is because such radon-proof membranes often result in levels of radon that exceed the level of action. Thus, Pacheco Torgal et al. [62] recommended ensuring that the radon barrier is adequately airtight at the building ground. This is achievable by making sure that feedthrough and joints are airtight and avoiding perforations. Similar to this recommendation, Arvela et al. [63] details the inclusion of elastic sealants and bitumen felt together with perforated pipe to lower the pressure of radon as a means to ensure such air tightness. In addition, Arvela et al. [63] indicated that the use of sub-slab piping coupled with an operating fan is efficient in preventing radon. This is because about 80 percent of the houses that had sub-slab piping and operating fans recorded concentration levels of radon below the 200 Bq/m3 action levels.
Groves Kirkby et al. [64] found that using the traditional sump technology alongside fans for remediation after construction was considerably effective in lowering the concentrations of radon on the one hand. On the other hand, installing radon-barrier membranes at construction did not offer enough protection from radon throughout. Specifically, this failed to lower the concentration levels of internal radon to less than the 200 Bq/m3 thresholds. However, Abdelouhab et al. [65] showed that Soil Depressurization Systems (SDS) is quite effective in lowering the concentrations of radon. However, mechanical SDS costs more than passive SDS.
The removal of fine particulates from air has also been discussed as a radon protection and reduction method. In an early study [66] it has been demonstrated that air cleaners had the capacity to reduce radon Decay Products (RDP) by between 72 and 89 percent. Later on, Yasouka et al. [67] showed that an air cleaner with filtration and one that has both filtration and carbon filter reduced RDP significantly. According to Larsso et al. [60], mechanical filtration that involves the use of console or portable air cleaning is also a viable option with potential for reducing exposure to radon [62]. This argument is in line with the findings by Hinds et al. [66], which showed that using a console fan reduced RDP by up to 64 percent whereas using ceiling fans achieved a 54 percent RDP reduction.
In a recent study, Blanco Rodríguez et al. [68] described the process of implementing systems to militate against radon in a radioactivity laboratory. They chose the positive ventilation solution, which comprised two fans blowing filtered, fresh air into the room where measurement was performed. They followed the approach of pressurizing to hinder the entry of radon and dilute it, as used by Sciyyer et al. [69]. They also installed air condition to work with the fans to enable the reduction of radon concentration and allow for repeat of conditions for the measurements. This resulted in a 76 percent reduction of radon concentration rendering enhanced positive ventilation a viable method for preventing exposure to radon through dilution and reduction [69]. 4 gaps and areas of further research.
Based on the above findings, it is clear that there are shortages and gaps in the research on radon in the several areas around the world such as The Gulf Countries Council (GCC). For instance, studies such as measurement of radon concentration in residential buildings and homes need to be conducted to measure the actual level of radon in those areas. In addition, a study conducted by Iglesias et al. [4] showed that tap water is major sources of radon; hence it is of critical importance that radon concentration in tap water should be evaluated. Furthermore, the exposure of radon at work or work-related radon exposure needs to be assessed. Over all, in the future studies it will be important to focus on the radon contaminated food and assess the relation between human exposures through contaminated food.
From the literature reviewed under the previous sections, there appears to contentions over the most effective approaches towards preventing or mitigating radon. However, the use of Soil Depressurization Systems whether passive or mechanical and the positive ventilation approaches seem to be the most preferred. Yet, as the study by Blanco Rodríguez et al. [68] shows, there is still room for advancing these two approaches. Evidently, all the research conducted on mitigating radon has been in the Western context. This means that the findings of such places are not generalizable or readily applicable to other geographic zones such as the Gulf region. This is because the concentration levels and density of radon have been shown to be affected by geological zoning and weather, which differ by location, as shown in this review. Although several radon mitigation measures have been fronted in literature, passive SDS and positive ventilation were found to be the most commonly used methods. The findings remain inconclusive as to the extent of effectiveness of the mitigation measures. Further research especially in the Gulf region is necessary to help contain its effects on public health.
This paper provided a review of previous theoretical, experimental, and empirical studies concerning radon indoor air pollution. Emphasis was made on the health impact of radon where it was identified as a significant contributor to lung cancer through its progenies and ranking only second to tobacco. Literature concerning domestic exposure to radon and childhood cancer was found to be inconsistent to prove the relation between the two. Moreover, it was established that different researchers use different methodological approaches such as case control and cohort data. Literature demonstrated that the main impacts of radon on the environment were on air quality and water contamination. In conclusion, exposure to radon and its progenies remains a significant challenge to date.
M. A. Contributed to writing the article and R. I. helped with technical input, reviewed and edited the content for final submission.
Competing financial interests: The authors declare no competing financial interests.
- National Research Council (1999) Health Effects of Exposure to radon. BEIR (Biological Effects of Ionizing Radiation) in National Academy Press p. 578. [Ref.]
- Petersen ML, Larsen T (2006) Cost-benefit analyses of radon mitigation projects. J Environ Manage 81: 19-26. [Ref.]
- W Roper (1999) “Toxicological profile for mercury. Agency for Toxic Substances and Disease Registry, USA. [Ref.]
- C Iglesias, J Taboada (2014) radon in Galicia. Procedia Earth Planet. Sci 8: 70-74. [Ref.]
- W. Bale (1951) Memorandum to the Files, Hazards associated with radon and thoron. Health Phys 38: 1062-1066. [Ref.]
- J H Harley (1953) Sampling and measurement of airborne daughter products of radon. Health Physics 11: 7. [Ref.]
- G RW Denton, Sara Namazi (2013) Indoor radon Levels and Lung Cancer Incidence on Guam. Procedia Environ. Sci 18: 157-166. [Ref.]
- WHO (2009) World Health Organization, WHO Handbook on indoor radon: A public health perspective. [Ref.]
- UNSCEAR (2006) United Nations Scientific Committee on the Effects of Atomic Radiation, Annex E - Sources-to-effects assessment for radon in homes and workplaces. [Ref.]
- ICRP (2012) International Commission on Radiological Protection. Annals of the ICRP 60. Compend. Dose Coefficients based ICRP Publ 40: 130.
- Barros-Dios JM, Ruano-Ravina A, Gastelu-Iturri J, Figueiras A (2007) Factors underlying residential radon concentration: results from Galicia, Spain. Environ Res 103: 185-190. [Ref.]
- Efstratios G. Vogiannis, Dimitrios Nikolopoulos (2014) radon Sources and Associated Risk in Terms of Exposure and Dose. Front Public Health 2: 207. [Ref.]
- J Chen, J Whyte, K Ford (2015) An Overview of radon Research in Canada. Radiat Prot Dosimetry 164: 1-5. [Ref.]
- López-Abente G, Núñez O, Fernández-Navarro P, Barros-Dios JM, Martín-Méndez I (2018) Residential radon and cancer mortality in Galicia, Spain. Sci Total Environ 610-611: 1125-1132. [Ref.]
- Gooding TD (2018) An analysis of radon levels in the basements of UK workplaces and review of when employers should test. J Radiol Prot 38: 247-261 [Ref.]
- Singh S, Kumar A, Singh B (2002) radon level in dwellings and its correlation with uranium and radium content in some areas of Himachal Pradesh, India. Environ Int 28: 97-101. [Ref.]
- Somlai J, Gorjánácz Z, Várhegyi A, Kovács T (2006) radon concentration in houses over a closed Hungarian uranium mine. Sci Total Environ 367: 653-665. [Ref.]
- Denman AR, Groves-Kirkby NP, Groves-Kirkby CJ, Crockett RG, Phillips PS, et al. (2007) Health implications of radon distribution in living rooms and bedrooms in U.K. dwellings--a case study in Northamptonshire. Environ Int 33: 999-1011. [Ref.]
- Masters G, Ela W (1991) Introduction to environmental engineering and science. Boston, USA. [Ref.]
- Kropat G, Bochud F, Jaboyedoff M, Laedermann JP, Murith C, et al. (2014) Major influencing factors of indoor radon concentrations in Switzerland. J Environ Radioact 129: 7-22. [Ref.]
- Collins SN (2013) Radionuclide Content of Sand Used for Construction in Kakamega County and Associated Indoor radon Diffusion Doses. Kenyatta University. [Ref.]
- Zielinski JM (2014) Mapping of Residential radon in the World. [Ref.]
- Magalhães MH, Amaral EC, Sachett I, Rochedo ER (2003) radon-222 in Brazil: An outline of indoor and outdoor measurements. J Environ Radioact 67: 131-143. [Ref.]
- Rangarajan S, Gopalakrishnan SS, Eapen CD (1974) The diurnal and seasonal changes in short-lived radon-thoron daughters’ concentrations in the coastal and inland regions of India and their possible relation to regional climatology. Pure and applied geophysics 112: 941-953. [Ref.]
- Zh HC, Charlet JM, Poffijn A (2001) radon risk mapping in southern Belgium: an application of geostatistical and GIS techniques. Sci Total Environ 272: 203-210. [Ref.]
- Kelsall DL (2015) Don’t ask, don’t tell: Canadian policies on radon. CMAJ 187: 635. [Ref.]
- US Environmental Protection Agency (2016) Health risk of radon. [Ref.]
- Gray A, Read S, McGale P, Darby S (2009) Lung cancer deaths from indoor radon and the cost effectiveness and potential of policies to reduce them. BMJ 338: a3110-a3110.
- Ahmad N, Ullah Khan I, Rehmana J, Nasir T (2017) An overview of radon concentration in Malaysia. J Radiat Res Appl Sci 10: 327-330. [Ref.]
- Almayahi BA,Tajuddin AA, Jaafar MS (2013) In situ soil 222Rn and 220Rn and their relationship with meteorological parameters in tropical Northern Peninsular Malaysia. Radiat Phys Chem 90: 11-20. [Ref.]
- Kumar A, Kaur M, Sharma S, Mehra R (2016) A study of radon concentration in drinking water samples of Amritsar city of Punjab (India). Radiat Prot Environ 39: 13-19. [Ref.]
- Akbari K, Mahmoudi J, Ghanbari M (2013) Influence of indoor air conditions on radon concentration in a detached house. J Environ Radioact 116: 166-173. [Ref.]
- Lantz PM, Mendez D, Philbert MA (2013) radon, smoking, and lung cancer: the need to refocus radon control policy. Am J Public Health 103: 443-447. [Ref.]
- Hevey D (2017) radon Risk and Remediation: A Psychological Perspective. Front Public Health 5: 1-5. [Ref.]
- Kim SH, Hwang WJ, Cho JS, Kang DR (2016) Attributable risk of lung cancer deaths due to indoor radon exposure. Ann Occup Environ Med 28: 8. [Ref.]
- Garzillo C, Pugliese M, Loffredo F, Quarto M (2017) Indoor radon exposure and lung cancer risk: a meta-analysis of case-control studies. Transl Cancer Res 6: 934-943. [Ref.]
- Zhang ZL, Sun J, Dong JY, Tian HL, Xue L, et al. (2012) Residential radon and lung cancer risk: an updated meta- analysis of casecontrol studies. Asian Pac J Cancer Prev 13: 2459-2465. [Ref.]
- Pavia M, Bianco A, Pileggi C, Angelillo I (2003) Meta-analysis of residential exposure to radon gas and lung cancer. Bull World Health Organ 81: 732-738. [Ref.]
- Lubin JH, Boice JD Jr (1997) Lung cancer risk from residential radon: meta-analysis of eight epidemiologic studies. J Natl Cancer Inst 89: 49-57. [Ref.]
- Raaschou-Nielsen O, Andersen CE, Andersen HP, Gravesen P, Lind M, et al. (2008) Domestic radon and childhood cancer in Denmark. Epidemiology 19: 536-543. [Ref.]
- Hauri D, Spycher B, Huss A, Zimmermann F, Grotzer M, et al. (2013) Domestic radon exposure and risk of childhood cancer: a prospective census-based cohort study. Environ Health Perspect 121: 1239-1244. [Ref.]
- Kendall GM, Little MP, Wakeford R, Bunch KJ, Miles JC, et al. (2013) A record-based case-control study of natural background radiation and the incidence of childhood leukaemia and other cancers in Great Britain during 1980-2006. Leukemia 27: 3-9. [Ref.]
- Peckham EC, Scheurer ME, Danysh HE, Lubega J, Langlois PH, et al. (2015) Residential radon Exposure and Incidence of Childhood Lymphoma in Texas, 1995-2011. Int J Environ Res Public Health 12: 12110-12126. [Ref.]
- Šenitková IJ, Šál J (2017) “Indoor radon Concentration Related to Different radon Areas and Indoor radon Prediction. Earth Environ Sci 95: 1-8. [Ref.]
- Šenitková I, Bucakova M (2004) radon and basement construction. In: proceedings of the 24th International Science Conference Industry Toxicology 113-116.
- Iranmanesh F, Bafti AS, Negarestani A, Malakootian M (2014) Estimated Annual Effective Doses of radon in Springs and Qanats Nearby Kouhbanan Active Fault System; Iran. J Sci Islam Repub Iran 25: 345-355. [Ref.]
- Erees FS, Aytas S, Sac MM, Yener G, Salk M (2007) radon concentrations in thermal waters related to seismic events along faults in the Denizli Basin, Western Turkey. Radiat Meas 42: 80-86. [Ref.]
- Gokhale B, Leung S (2010) Groundwater radon-222 Concentrations in Antelope Creek, Idaho: Measurement and Interpolation. Open Environ Biol Monit J 3: 12-20. [Ref.]
- Abdallah SM, Habib RR, Nuwayhid RY, Chatila M, Katul G (2007) radon measurements in well and spring water in Lebanon. Radiat Meas 42: 298-303. [Ref.]
- Namvaran M, Negarestani A (2013) Measuring the radon concentration and investigating the mechanism of decline prior an earthquake (Jooshan, SE of Iran). J Radioanal Nucl Chem 298: 1-8. [Ref.]
- Al-Nafiey MS, Jaafar MS, Bauk S (2014) Measuring radon concentration and toxic elements in the irrigation water of the agricultural areas in Cameron Highlands, Malaysia. Sains Malaysiana 43: 227-231. [Ref.]
- Aswood MS, Jaafar MS, Salih N (2017) Estimation of radon Concentration in Soil Samples from Cameron Highlands, Malaysia. Int J Sci Technol Soc 5: 9-12. [Ref.]
- Aswood MS, Jaafar MS, Bauk S (2014) Measuring radon Concentration Levels in Fertilizers Using CR-39 Detector. Adv Materials Res 925: 610-613. [Ref.]
- Saidi SA, Jaafar MS, Razab MKAA, Rasat MSM, Ahmad MI, et al. (2016) Potential of Fabricated Light Foamed Concrete in Reducing radon from Building Material. Materials Science Forum 840: 427- 431. [Ref.]
- Singh P, Singh P, Saini K, Bajwa BS (2017) Radionuclide measurements along with exhalation study in subsoil of southeast Haryana, India. Environ Earth Sci 76: 332. [Ref.]
- Singh P, Sahoo BK, Bajwa BS (2016) Theoretical modeling of indoor radon concentration and its validation through measurements in South-East Haryana, India. J Environ Manage 171: 35-41. [Ref.]
- Saini K, Singh P, Singh P, Bajwa BS, Sahoo BK (2017) Seasonal variability of equilibrium factor and unattached fractions of radon and thoron in different regions of Punjab, India. J Environ Radioact 167: 110-116. [Ref.]
- Bajwa BS, Singh P, Singh P, Saini K, Singh S, et al. (2016) A follow-up study on indoor 222Rn, 220Rn their decay product concentrations in a mineralised zone of Himachal Pradesh, India. Radiat Prot Dosimetry 168: 553-560.
- Lichtenstein E, Boles SM, Lee ME, Hampson SE, Glasgow RE, et al. (2008) Using radon risk to motivate smoking reduction II: randomized evaluation of brief telephone counseling and a targeted video. Health Educ Res 23: 191-201. [Ref.]
- Larsson LS (2014) Risk-reduction strategies to expand radon care planning with vulnerable groups. Public Health Nurs 31: 526-536. [Ref.]
- Denman AR, Phillips PS, Tornberg R, Groves-Kirkby CJ (2005) Analysis of the individual health benefits accruing from a domestic radon remediation programme. J Environ Radioact 79: 7-23.
- Torgal FP (2012) Indoor radon: An overview on a perennial problem. Build Environ 58: 270-277. [Ref.]
- Arvela H (2001) Experiences in radon-safe building in Finland. Sci Total Environ 272: 169-174. [Ref.]
- Groves-Kirkby CJ, Denman AR, Phillips PS, Crockett RG, Woolridge AC, et al. (2006) radon mitigation in domestic properties and its health implications--a comparison between during-construction and post-construction radon reduction. Environ Int 32: 435-443. [Ref.]
- Abdelouhab M, Collignan B, Allard F (2010) Experimental study on passive Soil Depressurisation System to prevent soil gaseous pollutants into building. Build Environ 45: 2400-2406. [Ref.]
- Hinds WC, Rudnick SN, Maher EF, First MW (1983) Control of Indoor radon Decay Products by Air Treatment Devices. J Air Pollut Control Assoc 33: 134-136. [Ref.]
- Yasuoka Y, Ishikawa T, Tokonami S, Takahashi H, Sorimachi A et al. (2009) radon mitigation using an air cleaner. J Radioanal Nucl Chem 279: 885-891. [Ref.]
- Rodríguez PB, Serantes LAF, Pazos AO, Rolle JLC, de Cos Juez FJ, (2017) radon Mitigation Approach in a Laboratory Measurement Room. Sensors (Basel) 17: 1090. [Ref.]
- Scivyer C (2011) radon in the Workplace: A Guide for Building Owners and Managers. IHS BRE Press, Garston, UK, 1-69. [Ref.]
Download Provisional PDF Here
Article Type: REVIEW ARTICLE
Citation: Al Jassim M, Isaifan R (2018) A Review on the Sources and Impacts of Radon Indoor Air Pollution. J Environ Toxicol Stud 2(1): dx.doi.org/10.16966/2576-6430.112
Copyright: © 2018 Al Jassim M, et al. This is an open-access article distributed under the terms of the Creative Commons Attribution License, which permits unrestricted use, distribution, and reproduction in any medium, provided the original author and source are credited.
Publication history:
SCI FORSCHEN JOURNALS
All Sci Forschen Journals are Open Access