Figure 1: The reactor was a column with a 48 cm height and an inside diameter of 10 cm.
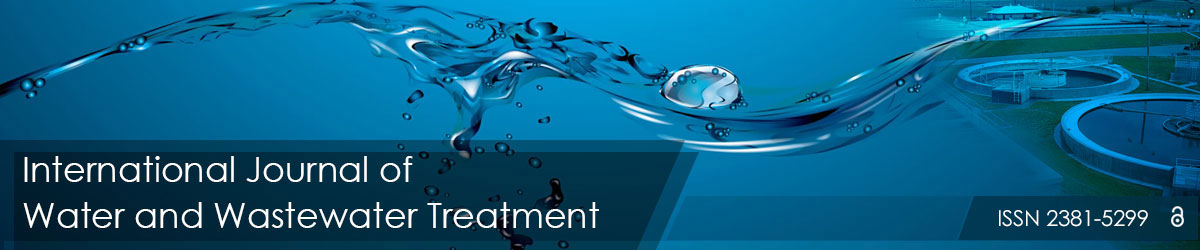
Full Text
Vongvisith Boualy Keobouala Chanthaboun Wang Changmei Yin Fang Wu Kai Yang Bin Yang Hong Zhang Wudi*
School of Energy and Environment Science, Yunnan Normal University, Kunming, China*Corresponding author: Zhang Wudi, School of Energy and Environment Science, Yunnan Normal University, Kunming, China, E-mail: wootichang@163.com
Nowadays, an anaerobic filter is used in treating various wastewaters that is more effective for denitrification and organic removal. The Anaerobic Filter with Inoculum, Soft and Hard Packing Medium (AFISH) and Double-Filling Aerobic Baffle (DFAB) reactors were continuously operated for 120 days with a short Hydraulic Retention Time (HRT) in the range of 1 and 2 days at an Organic Loading Rate (OLR) of 0.58 and 1.08 gCOD/(l.d) by daily feeding the influent Chemical Oxygen Demand (COD) concentration between 1169 and 250 mg/l. After treating the sample wastewater in the new AFISH reactor, the effluent flowed into the DFAB reactor for further treatment to determine the treatment effectiveness of the novel AFISH and DFAB reactors at a short HRT of 1 to 2 days. The influent COD was reduced to 376 mg/l to 250 mg/l in a novel AFISH effluent and further dropped to 220 mg/l to 102 mg/l following DFAB reactor treatment. The COD removal in the AFISH reactor was around 67% when HRT was about 1 day, and a high COD value of 78% was removed during the HRT of 2 days, while in the DFAB reactor, the capability of treatment reached between 40% and 57% of the COD removed at the HRT of 1 and 2 days. The concentration of NH4-N in the effluent of the novel AFISH reactor was significantly lower than the effluent of the DFAB reactor, with NH4-N concentrations ranging from 157 mg/l to 330 mg/l. However, the treatment of vegetable bio-slurry from the Internal Circulation (IC) reactor by the novel AFISH and DFAB reactors satisfied the secondary wastewater discharge standard in the Integrated Wastewater Discharge Standard. Therefore, this novel process is one method beneficial for current and further research development that can be applied for industrial wastewater treatment processing and provides science information to those interested in research in the future.
Anaerobic filter; Anaerobic digestion; Performance; Treatment effectiveness and treatment of vegetable biogas slurry
Anaerobic Digestion (AD) has been widely used to treat highstrength organic wastes and wastewater due to its potential for energy recovery and a useful technology in waste management and environmental health especially for mitigating Greenhouse Gases (G2HSs) [1]. An inherent drawback of AD is the slow growth of anaerobic microbes involved in the multi-stage process, particularly methanogens, leading to a low overall reaction rate [2]. Among the effective ways to overcome this limitation is using an attached-growth system where microorganisms are immobilized in biofilms to achieve high biomass concentration and long solids retention time [3]. AD is a promising and competent technology for treating various types of organic wastes and simultaneously producing biogas as a renewable energy carrier [4]. AD is a biological process that converts organic waste into biogas (55-70% CH4) by degrading the organic material into nutrient-rich digestate [5,6]. Currently, different kinds of anaerobic reactors have been developed for treating high protein concentration wastewater, such as the Up-Flow Anaerobic Sludge Blanket (UASB) [7], Expanded Granular Sludge Blanket (EGSB) [8], Anaerobic Filter (AF) [9,10], anaerobic Continually Stirred Tank Reactor (CSTR) [11], Anaerobic Membrane Bioreactor (AMBR) [12], and Anaerobic Filter Reactor (ABR) [13-15]. Nowadays, integrated biological treatment using an anaerobic aerobic system always works more efficiently in denitrification and organic removal of wastewater [16]. The Anaerobic Filter (AF) is a well-known attached growth process that has been widely used in the treatment of various wastewaters because of several advantages over aerobic process such as lower nutrient requirement, less surplus sludge production, and energy recovery via methane production [17,18]. Most of the anaerobic filter systems have been chosen because they work simply with energy-saving processes and avoid running costs for system installation. Nevertheless, the improvement of the Anaerobic Filter (AF) system to become more efficient in treating wastewater is challenging, compared to the traditional aerobic treatment system [19,20]. An Anaerobic Filter (AF) immobilizes activated microbes on the surface of the support media, enabling the reactor to withstand higher organic loading rates [10]. Using appropriate support media is important to the functioning of anaerobic filters as the physicochemical characteristics of the filter packing material have a significant influence on the attachment of biomass [10]. Anaerobic filters are an advantageous post-treatment option for septic tank effluent due to their technical and economic aspects: low operation and maintenance costs, operational simplicity, and low sludge production [21]. Anaerobic filters (AF) also have the advantage of allowing different packing materials to be used, and this fact is directly associated with implementation costs. An alternative to reducing costs is the replacement of synthetic packing materials with various regional materials, including ceramic bricks or grounded tires [22], bamboo rings [23] or green coconut husks [24], pebbles, plastic rings, granulated activated carbon, wooden blocks, and rubber sheets, which have been used to support the formation of biofilms in anaerobic filters [25]. Some studies on the effects of operating conditions such as hydraulic retention time and organic loading rate on anaerobic filter performance were reported [26,27]. And there are many researchers who have studied different anaerobic reactors used for energy recovery from vegetable waste and wastewater, including batch reactors, onestage reactors, and two-stage reactors. However, there seems to be limited research about the treatment of effluent from IC reactor treatment. As a result, a concept is being developed a novel process for the investigation of the performance and treatment effectiveness of the novel AFISH and DFAB reactors after treatment of vegetable juice waste from the IC reactor. AFISH operates in an upflow modes, and a variety of packing materials are used, such as sand, plastics, reticulated foam polymers, stone, granite, granular activated carbon, and quartz, that can be used as support materials in this process. The packing medium provides a mechanism for separating the solids and gas produced from the liquor. As expected, the achievements of this investigation will be the scientific guidelines and handbook for further industrial wastewater treatment. In the current study, a novel process was developed to determine the stability and assess the effectiveness of treatment of a novel Anaerobic Filter with Inoculum, Soft and Hard Packing Media (AFISH), and a Double-Filling Aerobic Baffle (DFAB) reactor at a short HRT of 1 to 2 days.
Experimental sample and inoculum
The effluent of the Vegetable Juice Waste (VJW) from the IC reactor, which was gathered after the IC reactor had been treated in the oven at 105°C for 5 hours for the determination of TS values, was transferred into a muffle furnace at 550°C for 2 hours for the calculation of the volatile solid and ash values, and had a Total Solid (TS), Volatile Solid (VS), and ash content of 3.9%, 92.0%, and 8%, respectively, was utilized as the sample wastewater (bio-slurry) used in the experiment in this paper. The sample wastewater had COD concentrations between 1146 and 1768 mg/l and NH4-N concentrations between 295 and 365 mg/l, as determined using the COD Max II and Amtax Compact II Ammonia Analyzers. After the initial treatment, the sample wastewater’s pH was determined to be 7.8 ± 0.2. The inoculum was obtained in the lab of the Bioenergy and Environment Engineering Research Group at the Solar Energy Research Institute, Yunnan Normal University, Kunming, P.R. China, after a year of anaerobic batch domestication. A new AFISH reactor was inoculated with seeding sludge by adding 0.39 ± 0.6l of adapted anaerobic granules to the reactor, which corresponds to 30% of the novel AFISH reactor’s working volume.
Experimental procedures
The new anaerobic filer reactor component consists of an inoculum, soft and hard packing medium (AFISH), which is cylindrical and made of plexiglass. The novel AFISH reactor has a total volume of 1.5l and an effective volume of 1.3l. The schematic diagram illustration of the novel AFISH reactor utilized in this experiment is presented in figure 1. The reactor was a column with a 48 cm height and an inside diameter of 10 cm. Table 1 shows that the AFISH and DFAB reactors were continuously operated for 120 days with a short HRT in the range of 1 and 2 days at an OLR of 0.58 and 1.08 gCOD/(l.d) by daily feeding the influent COD concentration between 1169 and 250 mg/l. After treating the sample wastewater in the new AFISH reactor, the effluent flowed into the DFAB reactor for further treatment. Figure 2 shows the schematic diagram of the DFAB reactor. Before the experiment began, the bio-slurry (sample wastewater) and inoculum were weighed, transferred into a crucible, and dried for five hours at 105°C to determine their Total Solids (TS), Volatile Solids (VS), and ash contents. Following TS estimation, the dried sample and ash were placed in a muffle furnace and heated to 550°C for two hours in order to calculate the volatile solid values. The sample wastewater’s influent and effluent COD and NH4-N concentrations were analyzed by using the Amtax Compact II online analyzer (Shanghai HACH Water Quality Analytical Instrument Co., COD Max II, China). The pH value was determined using pH paper. The temperature measurement used a portable electronic thermometer to measure the room temperature. A gas volume meter was used to measure daily biogas production, and the methane content was determined using gas chromatography (Zhejiang Fuli analytical Instrument Co., Fuli GC9790 II, China) equipped with a Thermal Conductivity Detector (TCD) and a Porapak Q packed column (stainless-steel 10’1/8”.085”) following the standard methods for the examination of water and wastewater by APHA [28].
Figure 2: The schematic drawing of the DFAB reactor.
Period | Influent COD | NH4-N | OLR | HRT | Daily feeding |
d | mg/l | mg/l | g COD/l.d | d | l/d |
1-60 | 1169 | 280 | 0.52 | 1 | 0.58 |
61-120 | 1136 | 391 | 1.08 | 2 | 1.23 |
Table 1: The operating conditions had set up in this experimental in both a new AFISH and DFAB reactors.
Changing of effluent COD during a novel AFISH reactor process when operated in the HRT of 1 and 2 days, respectively
After treatment in the IC reactor, Vegetable Juice Waste (VJW) effluent from the IC reactor was used as an influent sample for testing the performance and treatment effectiveness of a novel AFISH at hydraulic retention times of 1 and 2 days, respectively. Figure 3 shows the fluctuation in the curve of the influent and effluent COD concentrations and COD removal. When the influent COD varied from 1768 mg/l to 1716 mg/l, the effluent COD concentration in the first week dropped from 399 mg/l to 288 mg/l, and COD removal averaged around 80.4%. The average COD removal was roughly 67%, and the effluent COD concentrations varied from 673 mg/l to 226 mg/l. The influent COD concentration of the sample waste ranged from 1689 mg/l to 889 mg/l, with an average value of 1169 mg/l. During a novel AFISH reactor’s operating period for 0.5 gCOD/(l.d) of an OLR, the influent and effluent COD concentrations fluctuated under instability through the 60th day at the HRT of 1 day. Whereas the OLR in a novel AFISH reactor reached 1.08 gCOD/(l.d.) with the HRT for 2 days and daily sample feeding was approximately 1.23 l/d, there was a considerable COD removal of around 78% and a reduction in effluent COD concentration from 365mg/l to 173mg/l while the influent COD concentration was between 1070mg/l and 1023mg/l. A novel anaerobic filter with inoculum, soft, and hard packing medium (AFISH) reactor feeds sample wastewater from bottom to top (up-flow), where it comes into contact with the filter’s biomass and undergoes anaerobic degradation [29]. The efficiency of the Anaerobic Filter (AF) reactor for COD removal was approximately 79.6-95.3%, while for BOD it was 85%-91% [30]. According to the experimental results of this study, the effectiveness of COD removal was around 67% at an OLR of 0.5 g/(l.d) with an HRT of 1 day and 78% at an OLR of 1.08 g/(l.d) with an HRT of 2 days. While the pH for a new AFISH reactor was between 7.50.5- 8.50.5, the effluent wastewater from the IC reactor had a pH of around 6.8-7.5. The sample loading rate only had 0.5 and 1.08 gCOD/(l.d) to shock with the adaptation of the sludge microorganisms for growing during reactor operation, which was primarily responsible for the low microbial activity of microorganisms during the initial acclimatization stage, which may have contributed to the low pH condition.
Figure 3: The variation of the COD concentration during the AFISH reactor operation times.
The DFAB reactor efficiency treated sample wastewater (bioslurry) in the HRT of 1 and 2 days, respectively
The effluent wastewater COD content from a novel AFISH reactor filled the double-filling aerobic baffle (DFAB) for the treatment of bio-slurry, as shown in figure 4. With a COD removal efficiency of 32-12% (estimated to be 222-306 mg/l in the effluent), the removal of COD was initially low during the start-up (1-10 days) of the DFAB reactor. After adapting to the new inflow, the operation of the DFAB reactor was stable, and it achieved a slight removal of COD. The results of this study indicated that the HRT in the DFAB reactor dropped as the amount of bio-slurry (sample wastewater) entering the reactor increased steadily. Instability was seen in the effluent COD from the DFAB reactor, with values ranging from 376 mg/l to 220 mg/l in an HRT of 1 day. When the DFAB reactor was set up for 2 days of HRT, the effluent COD was observed, and the OLR increased from 0.5 to 1.08gCOD/(l.d), showing that it was similarly unstable at around 124 mg/l. However, throughout the DFAB reactor treatment from the 61st to the 120th day, the COD removed had simply reached 56.9%.
Figure 4: The variation of the COD concentration during the DFAB reactor operation times.
NH4-N concentration during the novel AFISH and DFAB reactors operated for 120 days
As shown in figure 5, the influent’s NH4-N concentration ranged from 268 mg/l to 370 mg/l in the first few days during operation and from 168 mg/l to 490 mg/l in the days that followed, from the sixth to the thirty-first. It was found that the influent NH4-N concentration varied along the graph line from the 31st to the 60th day when a 1day of HRT and an OLR of 0.5 gCOD/(l.d) were applied. The NH4-N in the influent varied from 302 to 471 mg/l throughout HRT 2 days, with an increase in OLR to 1.08 gCOD/(l.d). With an average of around 280 mg/l in HRT of 1 day and 391 mg/l in HRT of 2 days, the influent and effluent of the NH4-N curve lines showed instability in HRT of 1 and 2 days, respectively. The NH4-N curve lines’ influent and effluent showed changing instability in HRTs of 1 and 2 days, respectively, with average concentrations of roughly 280 mg/l in HRT of 1 day and 391 mg/l in HRT of 2 days. The concentrations of NH4-N in the effluent increased considerably when the OLR was raised from 0.5 to 1.08 gCOD/(l.d), increasing from 280 to 544 mg/l after one day of HRT to 391 to 571 mg/l after two days of HRT. Only a small amount of NH4-N is removed from the system, despite the possibility that some NH4-N may be absorbed by the growth of microorganisms. The stimulation of protein breakdown [31], which might result in the inorganic NH3-N being formed under anaerobic conditions [32], may be the cause of this rise in ammonium content. Similar to the above, the concentration of NH4-N in the influent and effluent of the wastewater sample graph lines revealed considerable unstable fluctuations during the HRT of the DFAB reactor for 1 and 2 days, respectively. According to figure 6, the concentrations of NH4-N in effluent increased significantly from 544 mg/l to 701 mg/l when the DFAB reactor was first operated from the first day to the 60th day at an HRT of one day, and increased from 571 mg/l to 901 mg/l when the HRT reached two days during inflow throughout the Double-Filling Aerobic Baffle (DFAB) reactor that was operated from the 61st day to the120th day. Following the consideration of the few concentrations of NO3 and NO2 in the reactor, the pathway for the reaction of anaerobic ammonium oxidation was greatly restricted [33-35]. In addition, the poisoning effect of NH3-N on the methanogen always occurred at an NH3 -N level higher than 1500 mg/l. As a consequence, the demerit of the increased NH3-N level on the bacteria was negligible. However, the C/N (measured in BOD/TN) in effluent was calculated to be 2:1 as more organic nitrogen was decomposed into the inorganic NH3-N. This would consistently increase the level of difficulty in subsequent aerobic bio-treatment, which was optimal at a C/N of 20:1 [36]. The pH of the DFAB reactor was between 8.5 ± 0.5 and 9.0 ± 0.5 throughout its 120 days of operation. Ammonia is included free ammonia (NH3) and its ionized form, ammonium (NH4 +), originates from the degradation of proteins, peptides, and amino acids. It is an important source of nitrogen for the growth of biogas-producing microorganisms [5]. Zuo Z, et al. [31] reported that the NH4+-N concentration of reactors gradually increased from approximately 700 mg/l to 1400 mg/l. Although some NH4+-N may be consumed by the growth of microorganisms, only small amounts of NH4+-N are removed from the system. This increase in ammonium concentration could be the result of the stimulated hydrolysis of proteins, which may be enhanced by increasing the Recirculation Rate (RR). According to He Q, et al. [37] who studied the treatment of soybean protein wastewater by a pilot-scale IC-A/O coupling reactor that was reported in the A/O system, NH3-N that was derived from the soybean protein could be converted into NO3 and NO2 through the nitration reaction in the A/O system, and then the nitrated reflux in the A system was substantially denitrified in a short HRT. In addition, the removal of COD by the aerobic microorganisms in the A/O system was also greatly strengthened, although the HRT was low. The high removal of NH3-N and COD in a short HRT was heavily reliant on the high microbial activity of mature microorganisms acclimated during the steady start-up stage [38]. Furthermore, this high removal rate was partially attributed to the reflux in the A/O system.
Figure 5: The concentration of NH4 -N in the influent and effluent of a new AFISH reactor operation at the HRT of 1 and 2 days.
Figure 6: The concentration of NH4-N in the influent and effluent of a new DFAB reactor operation at the HRT of 1 and 2 days.
Comparing the changes in effluent COD and COD removal between novel AFISH and DFAB reactors with different HRT
From the 1st day to the 120th day after the start-up of the novel AFISH and DFAB reactors, the experiment’s results in table 2 show comparable outcomes. The COD concentration in the effluent of the DFAB reactor was unstable, decreasing volumes in the range of 376mg/l to 220mg/l at HRT of 1 day by 40% of COD removal from the 1st day to the 60th day of reactor operation, while the DFAB reactor was set up for 2 days of HRT with the OLR increasing from 0.5 to 1.08gCOD/(l.d) that was also unstable at about 124 mg/L below. But the COD removed had just reached 57% during DFAB reactor treatment from the 61st to the 120th day. The NH4-N in effluent ranged from about 544 mg/l to 701 mg/l in the first few days of starting up the DFAB reactor at an HRT of 1 day and increased gradually from 571mg/l to 901mg/l when the HRT was 2 days. From the results of a new AFISH and DFAB reactor, it could be observed that from 1 day to 120 days after the starting up of a new AFISH reactor, the high concentration of COD in the influent was between 1169 mg/l and 146 mg/l, which was decreased from 376 mg/l to 250 mg/l in the novel AFISH effluent, and it was further decreased to 220 mg/l to 102 mg/l after the DFAB reactor treatment. While the COD removal was about 67% when the reactor operated with the HRT of 1 day, the high value of COD removal was 78% in the HRT of 2 days with a working OLR of 1.08 gCOD/(l.d.) that was 11% higher than 1 day of HRT in a novel AFISH reactor operating from 61 days to 120 days. In contrast, the concentration of NH4 -N in the effluent of the novel AFISH reactor was about 544 mg/l and 571 mg/l which was 157 mg/l to 330 mg/l less than the effluent of the DFAB reactor, where the effluent was about 701 mg/l to 901 mg/l. Ammonia, including free ammonia (NH3) and its ionized form, ammonium (NH4+), originates from the degradation of proteins, peptides, and amino acids. It is an important source of nitrogen for the growth of biogas-producing microorganisms [39]. Each group of microorganisms has a different optimal pH range. Methanogenic archaea can function within a very narrow pH range, with the optimal level being 6.5-8.0. Fermentation bacteria can function within a wider pH range of 4-8.5 [40]. The pH level also affects the acid-base equilibrium in the digester. In a mixedculture anaerobic digester, the optimal pH range is 6.8-8.0 [41]. In this study, the pH value was in the range of 7.5 ± 0.5-8.0 ± 0.5 in a novel AFISH, and the DFAB reactors were in the range of 8.5 ± 0.5-9.0 ± 0.5.
Reactor types | HRT | OLR | Influent COD | Effluent COD | COD removal |
Influent NH4-N |
Effluent NH4-N |
d | gCOD/l.d | mg/l | mg/l | % | mg/l | mg/l | |
AFISH | 1 | 0.58 | 1169 | 376 | 67 | 280 | 544 |
2 | 1.08 | 1146 | 250 | 78 | 391 | 571 | |
DFAB | 1 | - | 376 | 220 | 40 | 544 | 701 |
2 | - | 250 | 102 | 57 | 571 | 901 |
Table 2: Comparison of the effluent COD and COD removal between the new AFISH and DFAB reactors.
The experiment was conducted to investigate the stability of novel AFISH and DFAB reactors at a short HRT of 1 and 2 days. It was found that the high concentration of COD in influent ranged from 1169 mg/l to 1146 mg/l, which was decreased to 376 mg/l to 250 mg/l in a novel AFISH effluent and further decreased to 220 mg/l to 102 mg/l after the DFAB reactor treatment. The COD removal was about 67% when the reactor operated with the HRT of 1 day, and the high value of COD removal was 78% in the HRT of 2 days with the OLR at 1.08gCOD/(l.d) which was 11% higher than the HRT of 1 day. When the HRT was set up for 1 day, the influent COD decreased from 250 mg/l to 102 mg/l, and the capability of treatment reached 57% of COD removal, according to the DFAB reactor treated significantly. During this time, the COD removal rate was about 40%. The concentration of NH4-N in the effluent of the novel AFISH reactor was about 544 mg/l to 571 mg/l, which was 157 mg/l to 330 mg/l less than the effluent of the DFAB reactor, where the effluent was approximately 701 mg/l to 901 mg/l. However, the treatment of bio-slurry from the IC reactor by the novel AFISH and DFAB reactor processes satisfied the secondary wastewater discharge standard in the Integrated Wastewater Discharge Standard (GB89781996). Therefore, this novel process is one method beneficial for current and further research development that can be applied for industrial wastewater treatment processing and provides science information to those interested in research in the future. For further research, there should be a study on effective pH, HRT, and OLR in the AFSH and DAFB reactors.
I would like to express my gratitude to my supervisor, Wudi Zhang, for giving me the opportunity to do this experiment. Thanks for all the help and constructive comments and suggestions during this work. I also appreciate and thank all the Chinese friends who taught me a lot about laboratory work and helped me with all the problems that happened during the conducted experiment procedures.
This study was supported by the National Natural Science Foundation of China [51366015], the Yunnan Provincial Sciences and Technology Platform Promotion Plan [2013DH041], the Specialized Research Fund for Doctoral Program of Universities [20135303110001], the Yunnan Province Key Fund of Applied Basic Research [2014FA030], the Open Fund from Yunnan Key Laboratory of Rural Energy Engineering [2017KF03] and the China-Laos Joint Lab for Renewable Energy Utilization and Cooperative Development [2015DFA60120].
- Enitan-Folami AM, Swalaha FM (2021) In: Nasr M, Negm AM (eds) Cost-efficient Wastewater Treatment Technologies. The Handbook of Environmental Chemistry. Anaerobic Treatment System: A Sustainable Clean Environment and Future Hope of Renewable Energy Production. Springer 118: 169-198. [Ref.]
- Yan JQ, Lo KV, Pinder KL (1993) Instability caused by high strength of cheese whey in a UASB reactor. Biotechnol Bioeng 41: 700-706. [Ref.]
- Yee-Shian W, Tjoon-Tow T, Soon-An O, Norhashimah M, Rafatullah M, et al. (2014) Methane gas production from palm oil wastewater- An anaerobic methanogenic degradation process in continuous stirrer suspended closed anaerobic reactor. Journal Taiwan Institute Chemical Engineering 45: 896-900. [Ref.]
- de Lemos Chernicharo CA (2007) In: Biological wastewater treatment Series. Anaerobic reactors. London, IWA Publishing. [Ref.]
- Li Y, Park SY, Zhu J (2011) Solid-state anaerobic digestion for methane production from organic waste. Renewable Sustainable Energy Review 15: 821-826. [Ref.]
- Appels L, Van Assche A, Willems K, Degrève J, Van Impe J, et al. (2011) Peracetic acid oxidation as an alternative pre-treatment for the anaerobic digestion of waste activated sludge. Bioresour Technol 102: 4124-4130. [Ref.]
- Mainardis M, Buttazzoni M, Goi D (2020) Up-Flow Anaerobic Sludge Blanket (UASB) Technology for Energy Recovery: A Review on Stateof- the-Art and Recent Technological Advances. Bioengineering (Basel) 7: 43. [Ref.]
- Yang B, Wang M, Wang J, Song X, Wang Y, et al. (2018) Mechanism of high contaminant removal performance in the expanded granular sludge blanket (EGSB) reactor involved with granular activated carbon for low-strength wastewater treatment. Chemical Engineering Journal 334: 1176-1185. [Ref.]
- Rajakumar R, Meenambal T (2008) Start-up Performance of Anaerobic Filter (AF) Reactor Treating Poultry Slaughterhouse Wastewater, Nature Environment and Pollution Technology. Nature Environment and Pollution Technology 7: 219-224. [Ref.]
- Gao DW, Wen ZD, Li B, Liang H (2014) Microbial community structure characteristics associated membrane fouling in A/O-MBR system. Bioresour Technol 154: 87-93. [Ref.]
- Rincón B, Raposo F, Borja R, Gonzalez JM, Portillo MC, et al. (2006) Performance and microbial communities of a continuous stirred tank anaerobic reactor treating two-phases olive mill solid wastes at low organic loading rates. J Biotechnol 121: 534-543. [Ref.]
- Mulopo J (2017) Bleach plant effluent treatment in anaerobic membrane bioreactor (AMBR) using carbon nanotube/polysulfone nanocomposite membranes. Journal of Environmental Chemical Engineering 5: 4381-4387. [Ref.]
- Maynaud G, Druilhe C, Daumoin M, Jimenez J, Patureau D, et al. (2017) Characterisation of the biodegradability of post-treated digestates via the chemical accessibility and complexity of organic matter. Bioresour Technol 231: 65-74. [Ref.]
- Li R, Wu Z, Wang Y, Liu W (2014) Pilot study of recovery of whey soy proteins from soy whey wastewater using batch foam fractionation. Journal of Food Engineering 142: 201-209. [Ref.]
- Yu Y (2015) Research on soybean protein wastewater treatment by the integrated two-phase anaerobic reactor. Saudi J Biol Sci 22: 526- 531. [Ref.]
- Chowdhury P, Viraraghavan T, Srinivasan A (2010) Biological treatment processes for fish processing wastewater--a review. Bioresour Technol 101: 439-449. [Ref.]
- Neoh CH, Noor ZZ, Mutamim NSA, Lim CK (2016) Green technology in wastewater treatment technologies: Integration of membrane bioreactor with various wastewater treatment systems. Chemical Engineering Journal 283: 582-594. [Ref.]
- Lee MW, Lee HW, Joung JY, Park JM (2006) Modeling and simulation of anaerobic filter process: Two-dimensional distribution of acidogens and methanogens. studies in surface science and catalysis 159: 129-132. [Ref.]
- Loupasaki E, Diamadopoulos E (2013) Attached growth systems for wastewater treatment in small and rural communities: a review. Journal of Chemical Technology Biotechnology 88: 190-204. [Ref.]
- Abu Ghunmi L, Zeeman G, Fayyad M, van Lier JB (2010) Grey water treatment in a series anaerobic--aerobic system for irrigation. Bioresour Technol 101: 41-50. [Ref.]
- de Oliveira Cruz, Luana LM, Gomes BGLA, Tonetti AL, Figueiredo ICS (2019) Using coconut husks in a full-scale decentralized wastewater treatment system: The influence of an anaerobic filter on maintenance and operational conditions of a sand filter. Ecological Engineering 127: 454-459. [Ref.]
- Rajeshwari KV, Balakrishnan M, Kansal A, Kusum L, Kishore VVN (2000) State of the art of anaerobic digestion technology for industrial wastewater treatment. Renewable Sustainable Energy Review 4: 135-156. [Ref.]
- Barros AR, Adorno MA, Sakamoto IK, Maintinguer SI, Varesche MB, et al. (2011) Performance evaluation and phylogenetic characterization of anaerobic fluidized bed reactors using ground tire and pet as support materials for biohydrogen production. Bioresour Technol 102: 3840-3847. [Ref.]
- Camargo SA, Nour EA (2001) Bamboo as an anaerobic medium: effect of filter column height. Water Sci Technol 44: 63-70. [Ref.]
- de Oliveira Cruz LM, Stefanutti R, Filho BC, Tonetti AL (2013) Coconut shells as filling material for anaerobic filters. Springer Plus 2. [Ref.]
- Bodkhe S (2008) Development of an improved anaerobic filter for municipal wastewater treatment. Bioresource Technology 99: 222- 226.[Ref.]
- Merio-Solis ML, Villegas E, De Anda J, López-López A (2015) The effect of the hydraulic retention time on the performance of an ecological wastewater treatment system: an anaerobic filter with a constructed wetland. Water 7: 1149-1163. [Ref.]
- Clesceri LS, Greenberg AE, Eaton AD (1998) Standard methods for the examination of water and wastewater. 20th Edition, American Public Health Association. [Ref.]
- Rajagopal R, Torrijos M, Kumar P, Mehrotra I (2013) Substrate removal kinetics in high-rate upflow anaerobic filters packed with low-density polyethylene media treating high-strength agro-food wastewaters. J Environ Manage 116: 101-106. [Ref.]
- Morel A, Diener S (2006) Greywater Management in Low and Middle-Income Countries, Review of Different Treatment Systems for Households or Neighborhoods. Sandec at Eawag. [Ref.]
- Zuo Z, Wu S, Zhang W, Dong R (2014) Performance of two-stage vegetable waste anaerobic digestion depending on varying recirculation rates. Bioresour Technol 162: 266-272. [Ref.]
- Sasse L (1998) Decentralised wastewater treatment in developing countries. Bremen: Bremen Overseas Research and Development Association (BORDA). [Ref.]
- Li D, Chen L, Liu X, Mei Z, Ren H, et al. (2017) Instability mechanisms and early warning indicators for mesophilic anaerobic digestion of vegetable waste. Bioresource Technology 245: 90-97. [Ref.]
- Dong H, Zhang K, Han X, Du B, Wei Q, et al. (2017) Achievement, performance and characteristics of microbial products in a partial nitrification sequencing batch reactor as a pretreatment for anaerobic ammonium oxidation. Chemosphere 183: 212-218. [Ref.]
- Cai M, Wilkins D, Chen J, Ng SK, Lu H, et al. (2016) Metagenomic Reconstruction of Key Anaerobic Digestion Pathways in Municipal Sludge and Industrial Wastewater Biogas-Producing Systems. Front Microbiol 7: 778. [Ref.]
- Gu C, Zhou H, Zhang Q, Zhao Y, Di H, et al. (2017) Effects of various fertilization regimes on abundance and activity of anaerobic ammonium oxidation bacteria in rice-wheat cropping systems in China. Sci Total Environ 599-600: 1064-1072. [Ref.]
- He Q, Zhou J, Wang H, Zhang J, Wei L (2016) Microbial population dynamics during sludge granulation in an A/O/A sequencing batch reactor. Bioresour Technol 214: 1-8. [Ref.]
- Guo K, Shang Y, Gao B, Xu X, Lu S, et al. (2018) Study on the treatment of soybean protein wastewater by a pilot-scale IC-A/O coupling reactor. Chemical Engineering Journal 343: 189-197. [Ref.]
- Zhang X, Li L, Guo Y, Liu D, You T (2016) Amorphous flower-like molybdenum-sulfide-@-nitrogen-doped-carbon-nanofiber film for use in the hydrogen-evolution reaction. J Colloid Interface Sci 472: 69-75. [Ref.]
- Li N, Xue Y, Chen S, Takahashi J, Dai L, et al. (2017) Methanogenic population dynamics regulated by bacterial community responses to protein-rich organic wastes in a high solid anaerobic digester. Chemical Engineering Journal 317: 444-453. [Ref.]
- Hwang MH, Jang NJ, Hyun SH, Kim IS (2004) Anaerobic bio-hydrogen production from ethanol fermentation: the role of pH. J Biotechnol 111: 297-309. [Ref.]
Download Provisional PDF Here
Article Type: RESEARCH ARTICLE
Citation: Boualy V, Chanthaboun K, Changmei W, Fang Y, Kai W, et al. (2023) Research and Development of the Treatment Effectiveness of a Novel Process for Vegetable Biogas Slurry Purification. Int J Water Wastewater Treat 9(2): dx.doi.org/10.16966/2381-5299.190
Copyright: © 2023 Boualy V, et al. This is an open-access article distributed under the terms of the Creative Commons Attribution License, which permits unrestricted use, distribution, and reproduction in any medium, provided the original author and source are credited.
Publication history:
SCI FORSCHEN JOURNALS
All Sci Forschen Journals are Open Access