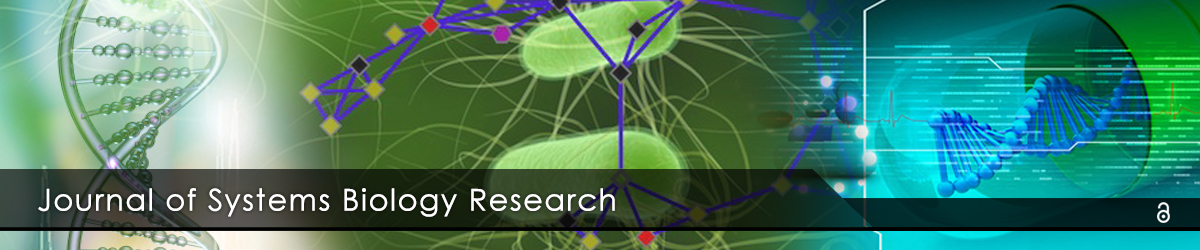
Full Text
Digvijay Verma1 Kavita Vasdev2*
1Department of Environmental Microbiology, Babasaheb Bhimrao Ambedkar University, Lucknow, India2Department of Microbiology, Gargi College, University of Delhi, New Delhi, India
*Corresponding author: Dr. Kavita Vasdev, Department of Microbiology, Gargi College, University of Delhi, New Delhi, India, E-mail: atvikav@gmail.com
Research into the human microbiome has led to our realization that the human body is a hub of trillions of microorganisms and most of the microbes that inhabit our body supply a crucial environment that benefits the entire host-microbe system. Several milestone discoveries have been carried out to understand the role of their existence in and on the human surfaces. The indigenous microbiota of healthy humans has a specific phylogenetic and functional context. Perturbations in microbial communities lead to dysbiotic conditions that come out with the onset of several non-infectious polymicrobial diseases. Present findings of the implication of microbiota are encouraging and pave a way to understand the insights of human microbiota especially in human healthcare, where several antibiotics are being degraded by microorganisms by adapting several mechanisms to cope up with their deleterious effect. This review provides an overview into the human microbiome, various approaches to study the microbiome, its significance, associated challenges with the human health and further research needed to exploit the potential of the human microbiota.
A human body harbors approximately one trillion of microorganisms in and on the surfaces, and their count shares the same number of cells as an average adult body [1]. Nobel laureate Joshua Lederberg introduced the term ‘Microbiome’ to understand the ecological community of inhabitant commensals, symbionts and pathobionts [2]. These indigenous microorganisms co-evolve and co-exist at different anatomical sites with distinct communities. Therefore, humans are now considered as ‘Superorganisms’, where microorganisms interact with the human cells and may impact their physiology, metabolism and immune responses in a harmonious relationship [3]. These commensal microorganisms include bacteria, fungi, viruses, and protozoa; which make the integral constituents of the microbiome; a well-established terminology dedicated for the total number of microorganisms, metagenomes and their interactions within a biological system. Bacteria comprise the major chunk of the microbiome and therefore designated as a new term ‘Bacteriome’ [4]. With the initiative of the National Institute of Health (NIH), the Human Microbiome Project (HMP) (www. hmpdacc.org/hmp) was founded in 2008 for exploring the inhabitant microorganisms of a human body; the 16S rRNA and shotgun sequencing revealed more than 14.23 terabytes of sequenced data, which is now publically available [5]. In this investigation, samples from various human body parts like skin, gut, urogenital tract, oral cavity and nasal passages were collected from three hundred individuals. Studies revealed that the intestinal microbiota contains the largest number of microorganisms followed by the oral cavity. Metagenomics of the Human Intestinal Tract (MetaHIT) (www.metahit.eu) and Human Oral Microbiome Database (HOMD) (www.homd. org) are another two established databases that deal with the human microbiome. The MetaHIT project was established to understand the effect of the gut inhabitant microorganisms on the health of humans. Specifically, it focuses on two disorders - IBD and obesity. The gut microbiota of a healthy individual chiefly harbors Bacteroides, Bifidobacterium, Enterococcus, Escherichia coli, Enterobacter, Staphylococcus, Lactobacillus, Clostridium and Salmonella [6].
The HOMD is founded by National Institute of Dental and Craniofacial Research during 2010 for maintaining the data of culturable and non-culturable microorganisms of the human’s oral cavity. Presently, HOMD comprises 700 prokaryotic species having 68% cultivable and 32% non-cultivable taxa [7].
Analyzing the microbial diversity of a niche is a big challenge, as the sample contains billions of microorganisms. Traditional microbiological methods are not adequate to access the entire microbial community of a niche due to the improper synchronization of several physiological and chemical parameters involved in cultivating them [8]. With the best of microbiologist’s efforts, only 0.1-1% of microorganisms can be cultivated in laboratory conditions [9,10]. Therefore, an alternate technology, metagenomics has been developed, which has nothing to do with the nourishment of microbes, rather it directly deals with the cloning of community DNA followed by their sequence and functional based analysis [11,12]. This non-traditional methodology starts with the extraction of good quality, non-sheared and high molecular weight DNA. Various phylogenetic molecular markers like bacterial/archaealspecific 16S rDNA and fungal-specific inter transcribed spacer (ITS) regions, that can be used for identifying the respective microbial diversity [13]. With the advent of next-generation sequencers, it is now possible to generate massive quantity of sequenced data from several samples simultaneously. Roche is the pioneer in the next generation sequencing (NGS) which is based on the principle of pyrosequencing and provides approximately 400-700 bp reads [14]. In the past five years, more efficient sequencing platforms viz., Illumina’s Hiseq and Miseq, ThermoFisher’s Iontorrent, and Oxford Technologies’ Nanopore were emerged that replaced the Roche technology [15]. NGS based platforms sequence only small stretches of 16S rDNA regions like V1-3 (464 bp), V3-4 (344 bp), V4-6 (474 bp) and V7-9 (348 bp) to amplify the hypervariable regions [16,17]. Human DNA contamination is a big challenge for studying the various pathways using shotgun based sequencing because it adds unnecessary sequenced data, however amplicon based sequencing does not demand for human DNA free metagenome and works well with various sets of primers [18]. For example, NGS based ribotyping is an excellent tool to characterize the microbial profiling to understand the microbial ecology of a niche using amplicon based sequencing [18,19]. Several modified methods have been reported where prior removal and selective eukaryotic cell lysis have been employed for reducing human DNA contamination [20]. NEBNext® Microbiome DNA Enrichment Kit (New England Biolabs, England), takes advantage of methylated DNA for its selective entrapment, leaving non-methylated bacterial DNA [21].
Massive sequencing of microbiome-based studies produces an enormous amount of data. Bacterial identification relies on 16S rRNA database like Greengenes, SILVA, and RDP, while functional identity is determined by MG-RAST, Pfam, TIGRfam, KEGG, SEED, CAGE and EBI databases. Several online tools are used for metagenome assembly and Gene calling and microbial diversity analysis (Table 1). Additionally, MEGAN, MG-RAST, and CAMERA can be used for comparative metagenomics (Table 1).
Alignment tools | ||
SILVA aligner | Provides aligned small (16S/18S, SSU) and large subunit (23S/28S, LSU) ribosomal RNA (rRNA) sequences | https://www.arb-silva.de/ |
Key DNA Tools | Utilizes short DNA stretches of 15 bp generated from reference core database | http://keydnatools.com/ |
Metagenome assembly | ||
Velvet | Algorithms for de-novo short reads assembly | https://www.ebi.ac.uk/~zerbino/velvet/ |
Metasim | Sequencing stimulator use to generate collection of synthetic reads and compare predictions | https://ab.inf.uni-tuebingen.de/ |
Euler | RepeatGluer algorithm to find all the sub-repeats in a genomic sequence | http://nbcr.sdsc.edu/euler/ |
Ray-Meta | For parallel genome assembly and parallel sequencing | http://denovoassembler.sourceforge.net/ |
Gene Calling | ||
Metag Gene Mark | Provides accesses to gene prediction in metagenomes and gene prediction program | exon.gatech.edu/meta_gmhmmp.cgi |
Glimmer-mg | Gene prediction in metagenomic sequences augmented by phylogenetic classification and clustering |
http://www.cbcb.umd.edu/software/ glimmer-mg/ |
Meta Gene Annotator | Detecting Species-Specific Patterns of Ribosomal Binding Site for Precise Gene Prediction in unknown genomes |
metagene.cb.k.u-tokyo.ac.jp/ |
Orphelia | ORF finding tool for predicting the protein coding genes in metagenomic sequences | orphelia.gobics.de/ |
Metagene | Diagnosis of inborn errors of metabolism | www.metagene.de/ |
Microbial diversity analysis | ||
MLST | Characterization of the unambiguous isolates of bacteria and other organisms using nucleotide approaches | www.mlst.net/ |
MOTHUR | Analyzes 16S rRNA gene sequences in metagenome | https://www.mothur.org/ |
EstimateS | Computes a wide range of species richness estimators for sample-based abundance and incidence | http://viceroy.eeb.uconn.edu/estimates/ |
QIIME | An open-source bioinformatics tool for performing microbiome analysis from raw metagenomes | http://qiime.org/ |
PHACCS | A dedicated tool for estimating the structure and diversity of uncultured viral communities | https://phaccs.soft112.com/ |
Comparative metagenomics | ||
MEGAN | Algorithms for functional analysis | ab.inf.uni-tuebingen.de/software/megan/ |
MG-RAST | SEED-based environment that allows users to upload metagenomes for automated analyses | https://metagenomics.anl.gov/ |
Table 1: Online tools for microbial diversity analysis.
The significance of the human microbiome got an attention when the fecal microbiota of healthy individual was utilized for treating recurrent infection of Clostridium difficile [22,23]. Since then, several studies have been carried out to understand the role of the human microbiome in human health. These unseen lodgers communicate well by replenishing the normal microbiota to overcome the deleterious effects of the pathogens [23]. However, side-effects and associated challenges with microbiome therapy can not be ignored and needs multiple clinical trials to establish it as a therapeutic [24]. Several investigations have proven the significant influence of inhabitant microorganisms on human physiology, metabolism and immune responses [4]. Recent discoveries have proven the role of gut microbiota in digestion, fat storage, food habits, angiogenesis and obesity [2,25,26]. Each healthy individual exhibits a unique microbiota which can be categorized into invariant and variant microbiota. Invariant microbiome constitutes the core microbiome, which rarely varies with the external factors, while the variant one significantly changes [27]. Moreover, one body harbors multiple core microbiomes depending on their habitats like oral, gut, skin and vaginal core microbiomes [28-31]. A healthy microbiome provides a baseline for maintaining a balance between the microbe associated metabolism and human health. Perturbations in healthy microflora cause dysbiotic conditions, where uninvited microorganisms encroach the space of normal inhabitants and significantly disrupt the normal communications [32]. Several investigations have been carried out to understand the role of altered microbial profile in diseased conditions like diabetes [33,34], bacteremia [35], endocarditis [36], cancer [37] and preterm births [38]. In an interesting report, gut dysbiosis has been reported for the altered spectra of microbial enzymes that influence the post-translational modification of the proteins involved in autoimmune diseases [38,39].
More recently the viral metagenome (virome) has been studied in different environments. This work is technically more challenging because there is no reference point equivalent to the ubiquitous 16S rRNA genes found in prokaryotes. Nevertheless, different viromes are being characterized to identify the pathogens and bacteriophages present. Interesting results are already emerging, with over 50% of the DNA or RNA sequences [40]. Ultimately, it is expected that new insights into the virus-host interactions will become possible. For example, knowledge of viral ecology could be used for monitoring emerging infections or assessing water quality [41]. Fungi constitute the another interesting microorganisms of the human microbiome, where Candida, Saccharomyces, Aspergillus, Fusarium and Penicillium, share the major chunk of the human mycobiome [42].
Additionally, viruses and fungi also constitute the significant chunk of microbiome and their existence could be utilized for detecting several infectious diseases [41].
With the understanding that replenishment of disturbed microbiota with a healthy one can be used for treating a disease, several apex companies like EpiBiome (www.epibiome.com), Enterome Biosciences (www.enterome.fr), Vedanta Biosciences (www.vedantabio.com) and Kallyope (www.kallyope.com) jumped into the commercialization of human-associated microbiota for their use in therapeutics and diagnostics. Microbiome therapy could be an alternate of antibiotics, when several mechanisms have been adapted by microorganisms to cope up with these antimicrobials [43]. There are global efforts to characterize the human microbiome through large-scale international initiatives, including the US-NIH based HMP, the European Commission’s Metagenomics of MetaHIT and the Canadian Microbiome Initiative (CMI). However, while our understanding of the phylogenetic and functional context of the human microbiome is rapidly increasing, it is still based on very few cohorts, resulting in underestimated variations due to parts of the world being understudied and under-represented in the data. At present, the majority of microbiome based studies focus on bacteriome analysis, where significant virome and mycobiome are less explored that can unlock the new horizons in host-microbe interaction based research to fully exploit the potential of the human microbiome.
KV and DV are thankful to the Principal, Gargi college, University of Delhi for her continuous encouragement of the work presented here.
- Sender R, Fuchs S, Milo R (2016) Revised Estimates for the Number of Human and Bacteria Cells in the Body. PLoS Biol 14: e1002533. [Ref.]
- Kilian M, Chapple JLC, Hannig M, Marsh PD, Meuric V, et al. (2016) The oral microbiome-an update for oral healthcare professionals. Br Dent J 221: 657-666. [Ref.]
- Hira P, Sood U, Gupta V, Nayyar N, Mahato NK, et al. (2017) Human Microbiome: Implications on health and disease. Genome analysis and human health: 153-168. [Ref.]
- Blaser MJ (2014) The microbiome revolution. J Clin Invest 124: 4162-4165. [Ref.]
- Turnbaugh PJ, Ley RE, Hamady M, Fraser-Liggett C, Knight R, et al. (2007) The human microbiome project: Exploring the microbial part of ourselves in a changing world. Nature 449: 804-810. [Ref.]
- Thursby E, Juge N (2017) Introduction to the human gut microbiota. Biochem J 474: 1823-1836. [Ref.]
- Chen T, Yu WH, Izard J, Baranova OV, Lakshmanan A, et al. (2010) The Human Oral Microbiome Database: A web accessible resource for investigating oral microbe taxonomic and genomic information. Databases: baq013. [Ref.]
- Verma D, Kawarabayasi Y, Miyazaki K, Satyanarayana T (2013) Cloning, expression and characteristics of a novel alkalistable and thermostable xylanase encoding gene (Mxyl) retrieved from compost-soil metagenome. Plos ONE 8: e52459. [Ref.]
- Amman RI, Ludwig W, Schleifer KH (1995) Phylogenetic identification and in situ detection of individual microbial cells without cultivation. Microbiol Rev 59: 143-169. [Ref.]
- Sommer MOA (2015) Advancing gut microbiome research using cultivation. Curr Opin Microbiol 27: 127-132. [Ref.]
- Handelsman J, Rondon MR, Brady SF, Clardy J, Goodman RM (1998) Molecular biological access to the chemistry of unknown soil microbes: A new frontier for natural products. Chem Biol 5: 245-249. [Ref.]
- Handelsman J (2004) Metagenomics: application of genomics to uncultured microorganisms. Microbiol Mol Biol Rev 68: 669-685. [Ref.]
- Klappenbach JA, Dunbar JM, Schmidt TM (2000) 16S rRNA operon copy number reflects ecological strategies of bacteria. Appl Environ Microbiol 66: 1328-1333. [Ref.]
- Alcaraz LD, Ferre PB, Rubio RC, Romero HA, Simon-Soro A, et al. (2012) Identifying a healthy oral microbiome through metagenomics. Clin Microbiol Infect 18: 54-57. [Ref.]
- Ambardar S, Gupta R, Trakroo D, Lal R, Vakhlu J (2016) High throughput sequencing chemistry. Indian J Microbiol 56: 394- 404. [Ref.]
- Kumar PS, Brooker MR, Dowd SE, Camerlengo T (2011) Target region selection is a critical determinant of community fingerprints generated by 16S pyrosequencing. Plos ONE 6: e20956. [Ref.]
- Yang B, Wang Y, Qian PY (2016) Sensitivity and correlation of hypervariable regions in 16S rRNA genes in phylogenetic analysis. BMC Bioinfo 17: 135. [Ref.]
- Kumar J, Kumar M, Gupta S, Ahmed V, Bhambi M, et al. (2016) An improved methodology to overcome key issues in human fecal metagenomic DNA extraction. Gen Prot Bioinfo 14: 371-378. [Ref.]
- Haque MM, Bose T, Dutta A, Reddy CV, Mande SS (2015) CS-SCORE: Rapid identification and removal of human genome contaminants from metagenomic datasets. Genomics 106: 116-121. [Ref.]
- Hunter SJ, Easton S, Booth V, Henderson B, Wade WG, et al. (2011) Selective removal of human DNA from metagenomic DNA samples extracted from dental plaque. J Basic Microbiol 51: 442-446. [Ref.]
- Feehery GR, Yigit E, Oyola SO, Langhorst BW, Schmidt VT, et al. (2013) A method for selectively enriching microbial DNA from contaminating vertebrate host DNA. Plos ONE 8: e76096. [Ref.]
- van Nood E, Vrieze A, Nieuwdorp M, Fuentes S, Zoetendal EG, et al. (2013) Duodenal infusion of donor feces for recurrent Clostridium difficile. N Eng J Med 368: 407-415. [Ref.]
- Kang DW, Adams JB, Gregory AC, Borody T, Chittick L, et al. (2017) Microbiota transfer therapy alters gut ecosystem and improves gastrointestinal and autism symptoms: an open-label study. Microbiome 5: 10. [Ref.]
- Mimee M, Citorik RJ, Lu TK (2016) Microbiome therapeuticsAdvances and challenges. Adv Drug Deliv Rev 105: 44-54. [Ref.]
- Lerner A, Matthias T (2016) GUT-the Trojan horse in remote organs’ autoimmunity. J Clin Cell Immunol 7: 401. [Ref.]
- Lerner A, Neidhofer S, Matthias T (2017) The gut microbiome feelings of the brain: a perspective for non-microbiologists. Microorganisms 5: 66. [Ref.]
- Bik EM, Eckburg PB, Gill SR, Nelson KE, Purdom EA, et al. (2006) Molecular analysis of the bacterial microbiota in the human stomach. Proc Natl Acad Sci U S A 103: 732-737. [Ref.]
- Grice EA, Konh HH, Renaud G, Young AC, Comparative Sequencing Program, et al. (2008) A diversity profile of the human skin microbiota. Genome Res 18: 1043–1050. [Ref.]
- Zaura E, Keijser BJ, Huse SM, Crielaard W (2009) Defining the healthy ‘core microbiome’ of oral microbial communities. BMC Microbiol 9: 259. [Ref.]
- Fierer N, Lauber CL, Zhou N, McDonald D, Costello EK, et al. (2009) Forensic identification using skin bacterial communities. Proc Natl Acad Sci USA 107: 6477–6481. [Ref.]
- Gevers D, Knight R, Petrosino JF, Huang K, McGuire AL, et al. (2012) The Human Microbiome Project: A community resource for the healthy human microbiome. PloS Biol 10: e1001377. [Ref.]
- Li J, Zhao F, Wang Y, Chen J, Tao J, et al. (2017) Gut microbiota dysbiosis contributes to the development of hypertension. Microbiome 5: 14. [Ref.]
- Genco RJ, Grossi SG, Ho A, Nishimura F, Murayama Y (2005) A proposed model linking inflammation to obesity diabetes and periodontal infections. J Periodontol 76: 2075-2084. [Ref.]
- Saremi A, Nelson RG, Tulloch-Reid M, Hanson RL, Sievers ML, et al. (2005) Periodontal disease and mortality in type 2 diabetes. Diabetes Care 28: 27-32. [Ref.]
- Poveda-Roda R, Jimenez Y, Carbonell E, Gavalda C, MargaixMunoz MM, et al. (2008) Bacteremia originating in the oral cavity : A review. Med Oral Patol Oral Cir Bucal 13: E355-E362. [Ref.]
- Parahitiyawa NB, Jin LJ, Leung WK, Yam WC, Samaranayake LP (2009) Microbiology of odontogenic bacteremia: Beyond endocarditis. Clin Microbiol Rev 22: 46-64. [Ref.]
- Michaud DS, Joshipura K, Giovannucci E, Fuchs CS (2007) A prospective study of periodontal disease and pancreatic cancer in US male health professionals. J Natl Cancer Inst 99: 171-175. [Ref.]
- Buduneli N, Baylas H, Buduneli E, Turkoglu O, Kose T, et al. (2005) Periodontal infections and pre-term low birth weight: A casecontrol study. J Clin Periodontol 32: 174-181. [Ref.]
- Lerner A, Aminov R, Matthias T (2016) Dysbiosis may trigger autoimmune diseases via inappropriate post-translational modification of host proteins. Front Microbiol 7: 84. [Ref.]
- Lerner A, Aminov R, Matthias T (2017) Transglutaminases in dysbiosis as potential environmental drivers of autoimmunity. Front Microbiol 8: 66. [Ref.]
- Mitreva M (2017) The Microbiome in infectious diseases. Infectious Diseases: 68-74.e2. [Ref.]
- Seed PC (2015) The Human Mycobiome. Cold Spring Harb Perspect Med 5: a019810. [Ref.]
- Lerner A, Matthias T, Aminov R (2017) Potential effects of horizontal gene exchange in the human gut. Front Immunol 8: 1630. [Ref.]
Download Provisional PDF Here
Article Type: MINI REVIEW
Citation: Verma D, Vasdev K (2018) Understanding The Potential of The Human Microbiome. J Sys Biol Res 1(1): dx.doi.org/10.16966/ jsbr.103
Copyright: © 2018 Verma D, et al. This is an open-access article distributed under the terms of the Creative Commons Attribution License, which permits unrestricted use, distribution, and reproduction in any medium, provided the original author and source are credited.
Publication history:
SCI FORSCHEN JOURNALS
All Sci Forschen Journals are Open Access