Scheme 1: Synthesis of 1-(2,4-dichloro benzylidene)-2,4-phenyl hydrazine
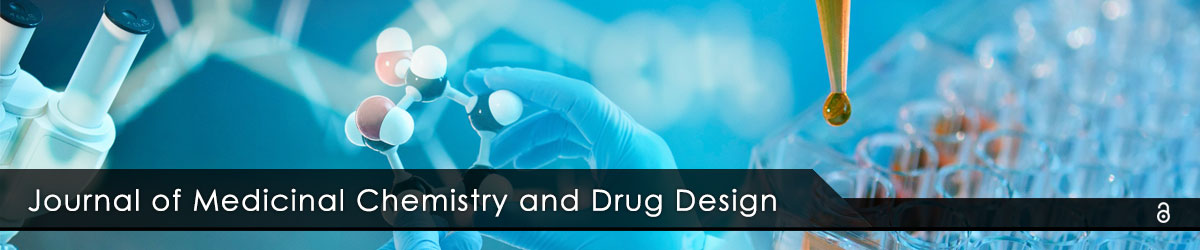
Full Text
Sodeeq Babalola1* Nosakhare Igie2 Isaiah Odeyemi2 Abdullahi Y Idris1 YM Sanni1 Asmau N Hamza1 Abdulrahman Bashir1 Umar Yakubu1 Salimat Sofela3 Thompson Odion Igunma4 Oladimeji S Olaluwoye5 Abdul Nafiu Bushirah Alaro6
1Department of Pharmaceutical and Medicinal Chemistry, Ahmadu Bello University, Nigeria2Department of Chemistry and Biochemistry, the University of Texas at Dallas, USA
3Department of Chemistry, Chrisland University, Nigeria
4Department of Material Science and Engineering, University of Florida, USA
5Department of Chemistry, Middle Tennessee State University, USA
6Faculty of Pharmacy, Ahfad University for Women, Sudan
*Corresponding author: Babalola Sodeeq Adewale, Department of Pharmaceutical and Medicinal Chemistry, Ahmadu Bello University, Zaria, Kaduna State, Nigeria, Tel: +2347058199796; E-mail: wale.babalola91@gmail.com
Drug-Drug Interactions (DDIs) has become an emerging and powerful strategy in drug designing and optimization. There has been an uptick in research during the past several years seeking to increase the therapeutic index of already marketed drugs with new chemical entity serving as actual activity enhancers as well as synergistic relationships. Here in, we report the synergistic drug-drug interactions of 1-(2,4-dichloro benzylidene)-2- phenyl hydrazine with piroxicam as an enhancer of efficacy showcasing faster onset of action with 48.09% inhibition and longer duration of action with 86.48% inhibition. This is compared to piroxicam’s moderate onset of action with 35.50% inhibition and shorter duration of action with 60.25% inhibition. 1-(2,4-dichloro benzylidene)-2-phenyl hydrazine molecule has also demonstrated potential increase in therapeutic index as a combination therapy with superior efficacy to NSAIDs-proton pump inhibitor combination therapy to manage inflammatory diseases.
Drug-drug interactions; Pharmacokinetic enhancer; Inflammation; Piroxicam; N-phenyl-2,4-dichlorophenyl hydrazone
Drug-Drug Interactions (DDIs) is the term that covers the results of combining two or more medications, including both desired and undesired consequences. Drug-Drug Interaction (DDI) studies are intended to identify how one drug may affect another’s metabolism and, vice versa. The results of DDI studies always fall into one of these two categories: (a) when a second drug increases the first drug’s catabolism, decreasing the first drug’s efficacy; or (b) when a second drug decreases the first drug’s catabolism, increasing the first drug’s efficacy (or increasing its toxicity) [1]. Drug interactions can occasionally be viewed as harmful and producing deleterious outcomes. However, in some circumstances, some are involved in the enhancement of treatments through combinatorial approach. This is achieved by boosting a pharmacological activity, addressing a known resistance mechanism, and blocking a metabolic route to improve the Pharmacokinetic (PK) profile of the main drug. Levodopa (L-Dopa) and dopadecarboxylase inhibitors constitute an instance. Other examples are clavulanic acid and β-lactam antibiotics, penicillin and probenecid, and 5-fluorouracil (5-FU) and folinic acid [2].
Lately, the glycogen synthase kinase inhibitor kenpaullone has been found to increase the action of temozolomide, an alkylating drug, in glioblastoma. This was accomplished by reducing stemcell features of glioblastoma cells while enhancing temozolomide’s apoptosis-inducing activity [2,3]. Another example is a microtubuledestabilizing drug, Carba1, a carbazole derivative, which increases the action of the antitumor drug, paclitaxel (Taxol) in tumor cells. The microtubules are stabilized by paclitaxel, however, Carba1’s alteration of tubulin dynamics promoted paclitaxel accumulation in the microtubules, increasing its efficacy [2,4].
Pharmacodynamic interactions, also known as pharmacodynamic DDIs, are DDIs that result from the combined pharmacodynamic effects of the interacting drugs [5]. One of these criteria is frequently used to select the second drug: (a) The two drugs are used to treat the same condition. Such is the case with the DDI trial involving the trial drug regorafenib and the second drug, irinotecan. When treating colorectal cancer, irinotecan and regorafenib are frequently co-administered. (b) The second drug is for a separate condition, co-administered with the trial drug, such as a contraceptive, acetaminophen, statin, a monoamine oxidase inhibitor, or warfarin. The condition that the second drug is intended to treat in this instance is different from that of the study drug [1].
Pharmacokinetic studies often involve a second drug. This relates to clinical pharmacokinetic trials that are used to predict which classes of drugs are most likely to participate in DDIs with the trial drug rather than to assess effectiveness or safety. In this case, the second drug is a recognized cytochrome P450 enzyme inhibitor and is typically employed as a prototype “second drug” in drug-drug inhibition research [1]. Example is the employment of ketoconazole in clinical investigations of DDIs as a model “second drug’. Ketoconazole inhibits cytochrome P450 3A4 when utilized in research on drugdrug interactions (CYP3A4). Although the medicine ketoconazole is technically an antifungal, this sort of clinical pharmacokinetic investigation that employs ketoconazole has no relation to the treatment of fungi [1]. Sometimes the activity and potency of a drug that has already been marketed are enhanced by its interaction with a single adjuvant molecule. Some drug-drug synergies have more recently been incorporated into a contemporary approach in drug design. These small molecules often referred to as enhancers, boosters, or activators, seldom or never have a direct impact on health. They can, however, enhance the action of a medicinal drug already in use, leading to greater therapeutic effects [2].
Pharmacokinetic (PK) Interactions (PK DDIs) sometimes called dispositional interactions is the modification of one drug’s PK or disposition (ADME) by another, which is the hallmark of these interactions. If these changes are severe enough, the exposure to the affected drug may change significantly, eventually modifying how the drug works [6]. In terms of PK, exposure is measured by the region enclosed by a drug’s plasma concentration versus time curve described as AUC. The pharmacological benefits which may be enhanced efficacy and/or undesirable adverse outcomes may manifest if the exposure (i.e., AUC) is greatly elevated. The therapeutic actions might not manifest if the exposure is sufficiently reduced, and therapeutic failure can result. Occasionally, variations in the peak plasma concentration (Cmax) of a drug influenced by another drug may be enough to have clinically significant negative effects [6].
The drug whose metabolism is affected is a “victim” drug, and the drug that modifies the disposition of a victim is a “perpetrator” drug in a DDI [6]. By reducing a victim drug’s metabolism in the liver or the intestines, preventing a victim drug’s efflux into the bile from hepatocytes, or improving its oral bioavailability, perpetrator drug may be anticipated to raise the victim drug’s AUC. This may occur if the perpetrator hindered the victim drug’s transporter-mediated efflux back into the lumen of the intestine from the intestinal epithelial cells, delayed its intestinal metabolism, lowered the victim drug’s protein or tissue binding, or its renal secretion into the urine [6].
On the other hand, it may be expected that a perpetrator will lower a victim drug’s AUC by, accelerating its metabolism in the liver or intestine, restricting its absorption or bioavailability, or by raising its efflux into the intestine’s lumen from intestinal cells or into bile from hepatocytes. Theoretically, the interference of perpetrator drug with which every aspect of victim drug’s ADME might change the victim drug’s AUC. This wholesome DDIs mechanistic view allowed for long-term prediction of several two-drug DDIs [5]. The vast majority of metabolic DDIs involve the inducers, inhibitors, and substrates of the Cytochrome P450 enzymes (CYPs). However, theoretically, any inducer or inhibitor of any drug-metabolizing enzyme might act as a perpetrator, and every substrate of any drug-metabolizing enzyme could act as a victim of DDIs. This is largely because CYPs predominate over all other types of enzymes in the metabolism of drugs [5].
Elvitegravir is a dramatic and unusual case since it is one of the few times when the package label calls for simultaneous administration of an inhibitor of CYP enzyme. The inhibitor of CYP enzyme was used to boost the study drug’s exposure. Another PK enhancer, cobicistat, is a CYP3A inhibitor thereby stops the degradation of certain drugs by CYP3A. For inhibiting CYP3A-mediated drug catabolism, ritonavir or cobicistat are referred to as helpful “pharmacokinetic boosters or enhancers” [1].
P-gp controls how well pharmaceuticals are absorbed through the intestines; therefore medicine that can stimulate or inhibit P-gp activity can lead to the development of DDI. The bioavailability of drugs that are ineffectively absorbed can be greatly increased by P-gp inhibition. It is known that sildenafil decreases Pgp’s transporter function, providing a potential method to increase the availability and perhaps even the effectiveness of anticancer drugs [7].
Drug interactions that affect distribution may be caused by interactions with drug transporters and displacement of drugs from plasma proteins, particularly serum albumin [8]. Displacement of one drug or substance by another drug or substance from its binding site or sites, which results in an increase in free drug concentration, is an example of a drug interaction of widespread clinical concern but unusual clinical importance. Higher distribution and clearance take place as a result of the increased free drug concentration, and the pharmacodynamic action is also improved [8]. In this situation, drugs that have a high affinity for plasma proteins, a small volume of distribution (Vd 1 L/kg), a prolonged half-life, and a low therapeutic index might have negative clinical consequences. The pharmacological displacement seen when warfarin and diclofenac are co-administered is a classic example. Because diclofenac and warfarin have a similar affinity for albumin, when it is given to a patient who is taking warfarin on a long-term basis, the warfarin is dislodged from its binding site. Serious hemorrhagic responses emerge as free warfarin concentrations in the plasma rise [9].
The drug’s elimination from the body might experience a variety of interactions in the organ from which it is expelled [10]. When two or more drugs employ the same transport route, DDI may result from a process of antagonism at the level of active tubular secretion. One example is how NSAIDs affect the amount of methotrexate toxicity when the anti-proliferative drug’s renal excretion is inhibited [11].
The interactions between the drugs can, however, be used therapeutically. For instance, probenecid can raise the blood levels of cephalosporins and penicillin, prolonging renal clearance and lowering dose requirements. The mechanism of action of probenecid is competitive inhibition, which increases other transporter substrates plasma concentrations while lowering clearance by interfering with an organic anion transporter in the renal tubules [12].
Inflammation is a biological defensive response that takes place when the body’s tissue homeostasis is upset by the presence of biological, chemical, or physical stimuli. Numerous pro-inflammatory mediators are released by the immune system, but when they are overproduced, as in chronic inflammation, it can lead to the emergence of a number of chronic disorders. Slowing down the inflammation process is therefore essential, and as a result, non-steroid anti-inflammatory drugs are frequently used at the risk of adverse effects [13].
The use of Non-Steroidal Anti-Inflammatory Drugs (NSAIDs) as antipyretics, analgesics, and anti-inflammatory treatments has spread around the world. In the United States, the market for NSAIDs was valued $5.78 billion in 2021 and projected to reach $7.10 billion by 2025. Global NSAIDs market was valued $15.58 billion in 2019, and it is expected to reach $24.35 billion by 2027 [14,15]. The increased incidence of conditions that cause inflammation and pain is one of the main factors propelling the market for NSAIDs [14]. We examined the anti-inflammatory activity of compound N-phenyl2,4-dichlorophenylhydrazone and it effect as efficacy enhancer in inflammation therapy in the current study
Chemistry
All of the chemicals used were obtained from Sigma Aldrich, and no additional purification was performed on them before use. Electrothermal Engineering LTD 9100 device was used to determine the melting points of the synthesized compounds. Brucker AMX 400 MHz spectrometer running at 400 MHz and 101 MHz was used to record the 1H and 13C NMR spectra respectively, Dimethyl Sulfoxide (DMSO) was utilized as the solvent. The NMR solvent peak is used as a reference point for chemical shifts (δ), which are given in parts per million. Agilent technologies spectrometer model 543 was used to record the FTIR spectra.
Synthesis: Equimolar proportions of phenylhydrazine (20 mmol) and 2,4-dichlorobenzaldehyde (20 mmol) were combined in 30 ml of ethanol at ambient temperature. For three hours, the mixture was continually stirred; the reaction’s development was used to track with TLC. The resulting white crystalline solid was collected by filter paper, allowed to dry, and then re-crystallized from pet ether.
Pharmacology Study
Experimental Animals: The experiment used 28 Swiss albino mice, weighing between 15 g and 34 g. The experimental animals were obtained in the Zaria neighborhood in Kaduna State, Nigeria. The mice were kept in same-sex cages in a room with a regulated condition (22 ± 2 °C and 50 ± 10% humidity) and on a 12-hour light: 12-hour dark cycle (lights on at 6 am). The mice were maintained for two weeks in order to stable, acclimate, and mature. At 7 weeks old, all the animals were adults and weighed between 15 g and 34 g. Water and the usual diet were freely available. The ABU Committee on Animal Use and Care’s bio-ethical committee protocols and the National Institutes of Health’s guidelines for the care and use of laboratory animals were followed in the performance of all animal experiments (NIH publication no. 85-23, revised 1985). The ABU Committee on Animal Use and Care was consulted for ethical permission, which it granted.
Oral Acute Toxicity Studies and lethality (LD50) test: Using the Fixed Dose Procedure (FDP)-OECD TG 420, the acute toxicity and Lethality (LD50) of the titled compounds was evaluated in mice. The OECD guidelines technique does not utilize an animal’s death as an obvious indicator of toxicity. Instead, any alterations such as in somatomotor activity, behavior patterns, eyes, mucosal membranes, the fur, skin, respiratory, autonomic, circulatory, and central nervous systems are considered. Attention is focused on signs of toxicity such as anesthesia, increased motor activity, tremors, arching and rolling, tonic extension, clonic convulsions, lacrimation, salivation, Straub reaction, muscle spasm, writhing, depression, loss of righting reflex, ataxia, blanching, stimulation, hypnosis, sedation, cyanosis, and analgesia, as well as sleeping, diarrhea, and lethargy [16].
The limit test was used in the experiment, and a group (n=3) was used for the test compound. The test animals were male Swiss albino mice, 20-25 g in weight. Aqueous suspension at a concentration of 50 mg/ml, or 2000 mg/kg for mice weighing 25 g, was created by mixing 150 mg of each titled compound with 3 ml of deionized water to provide the test dose. A dose of 2000 mg/kg (n=3) of the substance was given orally in the required volume of a 1% w/v deionized water suspension. After administration, animals were continually watched for any adverse symptoms during the first hour, as well as for the following 24 hours, 48 hours, 7 days, and 14 days.
Anti-inflammatory activity evaluation: Using a mouse model of carrageenan-induced hind paw edema, the named compound’s antiinflammatory efficacy was assessed. The method of Kasahara Y et al. [17], as described in our previous study [14].
Following the administration of the test compound, dosing vehicle, and reference drug, the thickness of the hind paw was measured using a thickness gauge vernier caliper both before each treatment (Vo) and after each interval (Vt). The data were represented using mean ± standard error of the mean (n=5). The data were statistically analyzed using Tukey’s Multiple Comparison Test, residual error test, and twoway Analysis of Variance (ANOVA) with replication. Calculating the percentage of swelling inhibition was done using the formula below:
Inhibition (%) = {[(Vt − Vo)control − (Vt − Vo)treated]/(Vt − Vo)control} × 100 Eq. (4)
Where Vt and Vo stand for the average volume of the mice’s hind paws prior to and following carrageenan injection, respectively. Each result was expressed using the mean ± Standard Error of the Mean (S.E.M.), and the percentage of inhibition.
In-silico pharmacokinetics (ADME) and toxicity studies: On Protox-II web (https://tox-new.charite.de) and ADMET lab 2.0 (https://admetmesh.scbdd.com), the in-silico pharmacokinetics, druglikeness, and toxicity studies were assessed.
Synthesis
1-(2,4-dichlorobenzylidene)-2-phenylhydrazine Yield 68.80%, Crystalline white solid, mp123-124 °C. FTIR (KBr, cm-1): 3302 (NH), 3030 (C-Himine), 1572 (C=N), 1517 (C=Caromatic), 1252 (C-N), 1047 (C-Cl). H1 NMR spectrum (400 MHz, DMSO-d6) δ, ppm: H1 NMR spectrum (400 MHz, DMSO-d6) δ, ppm: 7.01 d (1Harom, J= 7.1 Hz), 7.16 d (2Harom, 8.2 Hz), 7.22 d (2Harom, 7.8 Hz), 7.47 (Harom, 8.5 Hz), 7.63 (Harom, 1.7 Hz), 8.09 (Harom, 8.4), 8.19 (Himine), 11.23 (1H, NH). 13C NMR spectrum (101 MHz, DMSO-d6), δ, ppm: 112.30, 126.11, 126.54, 127.94, 128.62, 129.56, 131.89, 133.18, 134.34, 136.91, 139.89.
Twenty minutes after the start of the reaction white crystalline solids were produced with 68.80% yield after three hours according to results in table 1. The melting point was deduced to be 123-124 °C. N-H stretching, imine C-H stretching, and C=N stretching are characteristics of the FTIR absorption signals at 3302 cm-1, 3030 cm-1, and 1572 cm-1, according to spectroscopic investigation of the purified product. The hydrazone functional group’s synthesis in the molecule was verified by the singlet proton peaks’ locations at 8.19 ppm and 11.23 ppm as well as by the C-13 peak’s location at 136.91 ppm [18].
Table 1: Synthesis of N-phenyl-2,4-dichlorophenyl hydrazine.
Pharmacology
Oral Acute Toxicity Studies and Lethality:
(Table 2) The oral acute toxicity studies and LD50 of 1-(2,4-dichloro benzylidene)-2-phenyl hydrazine was evaluated at 2000 mg/kg dose in mice according to OECD guidelines. There was observed lethargy due to decreased motor activity and a modest feeling of tiredness as a sign of toxicity within the first hour of administration. This symptom wears off after the first our oral administration with no further sign of toxicity over the fourteen days of study. This analysis suggests a high relative safety and low chance of acute toxicity for oral delivery of the compound according to results in table 2. Furthermore, there was no mice death count observed during the fourteen days study, implying that the lethality dose of compound N-phenyl-2,4- dichlorophenylhydrazone is greater than 2000 mg/kg dose. By this, the LD50 of the compound 1-(2,4-dichloro phenyl)-2-phenyl hydrazine can be said to fall into Class V of the globally harmonized system (GHS) (2000mg/kg< LD50 ≤ 5000 mg/kg).
Compounds | Dose(mg/kg) | No. of Mice | Sign of toxicity | No. of dead mice |
Hydrazone | 2000 | 3 | Lethargy | None |
Table 2: Oral Acute Toxicity Studies and Lethality (LD50) results.
In-silico pharmacokinetics (ADME) and Anti-inflammatory activity evaluation results
1-(2,4-dichloro benzylidene)-2-phenyl hydrazine demonstrated statistically significant (p-value< 0.005) anti-inflammatory activity at all the tested doses in the carrageenan-induced paw edema in mice according to the results in table 7.
The test compound was observed to exhibit slow onset of action at 30 mg/kg and 50 mg/kg doses with percentage inhibitions of 17.94% and 26.72% after first one and half hour of inflammation induction. These are compared to the 35.50% and 33.21% inhibitions of paw edema of the reference drugs piroxicam and celecoxib respectively. The slow onset of action of compound 1-(2,4-dichloro phenyl)-2-phenyl hydrazine may be due to its pharmacokinetic profile [19] as observed in the results in tables 3-6. Though 1-(2,4-dichloro benzylidene)-2- phenyl hydrazine molecule exhibited good absorption in both MadinDarby Canine Kidney cells (MDCK), human colon adenocarcinoma cell lines (Caco-2), and human intestinal absorption (HIA) with 12.0×10-6 cm/s, -4.28 cm/s, 0.003 respectively in in-silico models which is better than that of piroxicam with -6.05 cm/s, 18.0×10-6cm/s, and 0.010 respectively as demonstrated in table 3. It high plasma protein binding (PPB) of 100.52% leads to its high distribution within tissues (Vd = 2.731 L/kg) with consequent low fraction unbound (Fu= 0.82%) thereby causing it low bioavailability, as 30% of the test compound is not bioavailable (F30%= 0.976) compared to piroxicam with proper PPB of 73.60%, volume distribution of 0.340 L/kg, Fu of 27.15%, and consequent high bioavailability (F30% = 0.003) table 4. This explains why 1-(2,4-dichloro benzylidene)-2-phenyl hydrazine exhibited slow onset of action and while piroxicam exhibited rapid onset of action accordingly.
Compounds | Caco-2 | MDCK | Pgp-inhi | Pgp-subs | HIA | F30% | F20% | F10% |
Hydrazone | -4.28 | 12.0 × 10-6 | 0.004 | 0.032 | 0.003 | 0.976 | 0.062 | 0.55 |
Piroxicam | -6.05 | 18.0 × 10-6 | 0.082 | 0.002 | 0.010 | 0.003 | 0.002 | 0.56 |
Table 3: Absorption.
Compounds | Plasma Protein Binding | Volume Distribution | BBB | Fraction Unbound |
Hydrazone | 100.52% | 2.731 L/kg | 0.198 | 0.82% |
Piroxicam | 73.60% | 0.340 L/kg | 0.967 | 27.15% |
Table 4: Distribution.
Compounds | CYP1A2 | CYP2C19 | CYP2C9 | CYP2D6 | CYP3A4 | |||||
Inhib. | Subs. | Inhib. | Subs. | Inhib. | Subs. | Inhib. | Subs. | Inhib. | Subs. | |
Hydrazone | 0.99 | 0.47 | 0.95 | 0.12 | 0.81 | 0.84 | 0.55 | 0.76 | 0.20 | 0.26 |
Piroxicam | 0.17 | 0.62 | 0.13 | 0.20 | 0.10 | 0.95 | 0.22 | 0.14 | 0.20 | 0.58 |
Table 5: Metabolism.
Compounds | Clearance (mL/min/kg) | Half-life (T1/2) |
Hydrazone | 5.697 | 0.152 |
Piroxicam | 1.033 | 0.561 |
Table 6: Excretion.
Test sample | 0 hr | 1hr 30min | 3hrs | 4hrs 30mins | 6hrs |
*10mg/kg | 1.30 ± 0.02 | 1.58 ± 0.02 | 1.61 ± 0.04 | 1.54 ± 0.04 | 1.37 ± 0.03 |
30mg/kg | 1.26 ± 0.04 | 1.69 ± 0.06 | 1.63 ± 0.02 | 1.54 ± 0.02 | 1.39 ± 0.01 |
50mg/kg | 1.29 ± 0.05 | 1.68 ± 0.06 | 1.67 ± 0.09 | 1.60 ± 0.03 | 1.54 ± 0.01 |
Piroxicam | 1.32 ± 0.03 | 1.66 ± 0.04 | 1.67 ± 0.04 | 1.53 ± 0.01 | 1.52 ± 0.02 |
Celecoxib | 1.27 ± 0.01 | 1.62 ± 0.04 | 1.66 ± 0.05 | 1.56 ± 0.02 | 1.52 ± 0.02 |
Normal saline | 1.27 ± 0.03 | 1.80 ± 0.05 | 1.87 ± 0.07 | 1.81 ± 0.04 | 1.76 ± 0.03 |
Table 7: Mean ± S.E.M of hind paw thickness at 10mg/kg dose. n=5.
*=Co-administration of 10 mg/kg each of the hydrazone and piroxicam.Mean ± S.E.M of hind paw thickness, n=5, p-value=0.00064 < α=0.05, F = 5.31 > F Critical=2.46
However, there is observed increase in onset of action as the dose was increased from 30mg/kg to 50mg/kg dose of compound N-phenyl-2,4-dichlorophenyl hydrazone suggesting possible increase in bioavailability. Also, 1-(2,4-dichloro benzylidene)-2-phenyl hydrazine exhibited progressive increase in activity over the hours of inflammation induction in carrageenan-induced paw edema in mice for each dose as indicated in table 8. Conversely, when the activity of 1-(2,4-dichloro benzylidene)-2-phenyl hydrazine was compared at these two doses, there was steady decrease in activity after the first three hours of inflammation induction as the dose increased from 30mg/ kg to 50mg/kg. This observed decrease in activity has been attributed to the high clearance rate (CL=5.697 mL/min/kg) of compound 1-(2,4-dichloro phenyl)-2-phenyl hydrazine, resulting in increased in clearance and reduced Fu as dose increases. This high clearance of compound 1-(2,4-dichloro benzylidene)-2-phenyl hydrazine compared to the rate of clearance of piroxicam (CL=1.033 mL/min/ kg) in table 6 can also be traced to it possible metabolism by CYP enzymes [19] in table 5. This can be accounted for by its possible early metabolism and contributing first-pass effect which is a consequence of its high lipophilicity [19], and generally limiting it bioavailability.
Test sample | 0hr | 1hr 30min | 3hrs | 4hrs 30min | 6hrs |
10mg/kg* | 0.00% | 48.09% | 47.97% | 56.41% | 86.48% |
30mg/kg | 0.00% | 17.94% | 37.84% | 47.58% | 72.54% |
50mg/kg | 0.00% | 26.72% | 36.82% | 43.49% | 50.00% |
Piroxicam | 0.00% | 35.50% | 42.06% | 55.41% | 60.25% |
Celecoxib | 0.00% | 33.21% | 34.80% | 46.84% | 49.18% |
Normal Saline | 0.00% | 0.00% | 0.00% | 0.00% | 0.00% |
Table 8: Percentage inhibitions of hind paw edema at 10mg/kg dose.
*=Co-administration of 10 mg/kg each of the hydrazone and piroxicam
Co-administration of 10 mg/kg dose of compound N-phenyl2,4-dichlorophenylhydrazoneand 10 mg/kg of piroxicam resulted in marked increase in onset of action of 48.09% compared to either of the two agents’ independent onset of action. Furthermore, the coadministered dose was superior in activity to celecoxib and piroxicam used as reference drugs with steady increase in activity over the hours of inflammation induction. More importantly, the co-administered dose demonstrated significant longer duration of action with 86.48% inhibition of paw edema compared 60.25% and 49.18% for piroxicam and celecoxib respectively. Though the mechanism associated with the enhanced efficacy has not been fully elucidation, however, the in-silico studies revealed that1-(2,4-dichloro benzylidene)-2-phenyl hydrazine is a stronger inhibitor of P-glycoprotein compared to piroxicam with 0.004 and 0.082 respectively as illustrated in table 3. Also, piroxicam is an inhibitor of cytochrome P450 metabolizing enzymes CYP1A2, CYP2C19, and CYP3A4 that are responsible for the metabolism of 1-(2,4-dichloro benzylidene)-2-phenyl hydrazine.
Therefore, the efficacy enhancements of the combinatorial approach maybe primarily due to pharmacokinetic drug-drug interactions (DDI). Furthermore, the longer half-life of 1-(2,4-dichloro benzylidene)-2-phenyl hydrazine (T1/2=0.152) compared to piroxicam (T1/2=0.561) as illustrated in table 6 suggests that 1-(2,4-dichloro benzylidene)-2-phenyl hydrazine will remain in circulation eliciting activity longer after piroxicam may have been excreted contributing to the longer duration of action of the combined dose. Therefore, the enhanced activity of the co-administered dose maybe due to increased inhibition of P-glycoprotein transporter and cytochrome P450 metabolizing enzymes leading to increased bioavailability and delayed metabolism and a consequent increased exposure to the target site. However, since the two agents are active against particular disease state, the observed efficacy is due to contributing activities of both compound N-phenyl-2,4-dichlorophenylhydrazone and piroxicam which explains the synergistic pharmacokinetic-pharmacodynamics DDIs.
Furthermore, 1-(2,4-dichloro benzylidene)-2-phenyl hydrazine have demonstrated significant antimicrobial activity in in-vitro assay [18] against wide range of bacteria and fungi strains including Helicobacter pylori which causes excessive secretion of gastrointestinal acid. It has also been demonstrated that 1-(2,4-dichloro benzylidene)-2-phenyl hydrazine efficiently inhibited proton pump enzyme H+/K+ ATPase showcasing plausible interaction with key amino acid CYS-822 [19,20]. Therefore, the use of compound N-phenyl-2,4-dichlorophenylhydrazone in combination therapy as a new design approach in the treatment of inflammatory diseases will lead to improved therapeutic index as a result of enhanced efficacy and diminished gastro-intestinal toxicity associated with piroxicam and other NSAID therapy. The enhanced efficacy is an advantage over the conventional NSAIDs-proton pump inhibitor combination therapy and, therefore, resultant increase in therapeutic index.
In this study, the synthesized 1-(2,4-dichloro benzylidene)-2- phenyl hydrazine has indicated potential as an activity enhancer through synergy with piroxicam indicating promise as an alternative therapy to conventional NSAIDs-proton pump inhibitor combination therapy with improved therapeutic index.
Sodeeq Babalola, Abdullahi Y Idris, Sanni YM, and Asmau N Hamza contributed in the concept development, design and method development.
Sodeeq Babalola, Abdul rahman Bashir, Umar Yakubu contributed in the laboratory experiment.
Sodeeq Babalola and Abdul Nafiu Bushirah Alaro contributed in results analysis.
Sodeeq Babalola, Nosakhare Igie, Isaiah Odeyemi, Salimat Sofela, Thompson Odion Igunma, and Oladimeji S Olaluwoye contributed in manuscript writing and review.
All authors declared no conflict of interest.
- Drug-Drug Interactions: Part One (Small Molecule Drugs) (2018) FDA’s drug review process and the package label.
- Guieu B, Jourdan JP, Dreneau A, Willand N, Rochais C et al. (2020) Desirable drug-drug interactions or when a matter of concern becomes a renewed therapeutic strategy. Drug Discov Today 26: 315-328. [Ref.]
- Kitabayashi T, Dong Y, Furuta T, Sabit H, Jiapaer S, et al. (2019) Identification of GSK3 β inhibitor kenpaullone as a temozolomide enhancer against glioblastoma. Sci Rep 9. [Ref.]
- Peronne L, Denarier E, Rai A, Prudent R, Vernet A, et al. (2020) Two Antagonistic Microtubule Targeting Drugs Act Synergistically to Kill Cancer Cells. Cancers 12: 2196. [Ref.]
- Campbell JE, Cohall D (2017) Pharmacodynamics-A Pharmacognosy Perspective. Pharmacog 513-525. [Ref.]
- Bachmann K (2009) Drug-Drug Interactions with an Emphasis on Drug Metabolism and Transport. Pharmacol 303-325. [Ref.]
- Shi Z, Tiwari AK, Shukla S, Robey RW, Singh S, et al. (2011) Sildenafil reverses ABCB1 and ABCG2 mediated chemotherapeutic drug resistance. Cancer Res 71: 3029-3041. [Ref.]
- Neely MN, Reed MD (2018) Pharmacokinetic-Pharmacodynamic Basis of Optimal Antibiotic Therapy. Princ Prac of Pediat Infect Dis 1478-1498. [Ref.]
- Palleria C, Di Paolo A, Giofrè C, Caglioti C, Leuzzi G, et al. (2013) Pharmacokinetic drug-drug interaction and their implication in clinical management. J Res Med Sci 18: 601-610. [Ref.]
- Kido Y, Matsson P, Giacomini KM (2011) Profiling of a prescription druglibrary for potential renal drug-drug interactions mediated by theorganic cation transporter 2. J Med Chem 54: 4548-4558. [Ref.]
- Kristensen MB (1976) Drug interactions and clinical pharmacokinetics. Clin Pharmacokinet 1: 351-372. [Ref.]
- Wu H, Liu M, Wang S, Feng W, Yao W, et al. (2010) Pharmacokinetic properties and bioequivalence of two compound formulations of 1500 mg ampicillin (1167 mg)/probenecid (333 mg): a randomized sequence, single-dose, open-label, two-period crossover study in healthy Chinese male volunteers. Clin Ther 32: 597-606. [Ref.]
- Ambriz-Pérez DL, Leyva-López N, Gutierrez-Grijalva EP, Heredia JB (2016) Phenolic compounds: Natural alternative in inflammation treatment. A Review. Food Sci Technol 2. [Ref.]
- Babalola S, Igie N, Odeyemi I, Idris AY, Sanni YM, et al. (2022) In-vivo anti-inflammatory activity studies of some p-nitrophenylhydrazones. Discovery 58: 698-706. [Ref.]
- Fortune Business Insight. Healthcare, 2020. Pharmaceutical. Global non-steroidal anti-inflammatory drugs (NSAIDs) market analysis, insights and forecast, 2016-2027.
- Paramveer SD, Chanchal KM, Paresh M, Rani A, Shrivastava B, et al. (2010) Effective alternative methods of LD50 help to save number of experimental animals. J Chem Pharm Res 2: 450-453. [Ref.]
- Kasahara Y, Hikino H, Tsurufuji S, Watanabe M, Ohuchi K (1985) Antiinflammatory actions of ephedrines in acute inflammations1. Planta Med 51: 325-331. [Ref.]
- Babalola SA, Igie N, Idris AY, Hamza A, Sanni YM (2022) Antimicrobial activities of hydrazones with 2, 4-dichloro moiety. Drug Discovery 16: 53-62. [Ref.]
- Babalola S, Igie N, Odeyemi I (2022) Structure-based discovery of multitarget directed anti-inflammatory p-nitrophenylhydrazones; molecular docking, drug-likeness, in-silico pharmacokinetics, and toxicity studies. [Ref.]
- Babalola S, Igie N, Odeyemi I (2022) Molecular Docking, Drug- Likeness Analysis, In-Silico Pharmacokinetics, And Toxicity Studies Of p-NitrophenylHydrazones As Anti-Inflammatory Compounds Against COX-2, 5-LOX And H+/K+ ATPase. Pharmaceut Fronts 4: 250- 266. [Ref.]
Download Provisional PDF Here
Download Provisional Supplementary Figures Here
Article Type: RESEARCH ARTICLE
Citation: Babalola S, Igie N, Odeyemi I, Idris AY, Sanni YM, et al. (2023) Synergistic Drug-Drug Interactions (DDIs) Effects on Anti-Inflammatory Activities of N-phenyl-2,4-Dichlorophenylhydrazone and Piroxicam. J Med Chem Drug Des 4(1): dx.doi.org/10.16966/2578-9589.120
Copyright: © 2023 Babalola S, et al. This is an open-access article distributed under the terms of the Creative Commons Attribution License, which permits unrestricted use, distribution, and reproduction in any medium, provided the original author and source are credited.
Publication history:
SCI FORSCHEN JOURNALS
All Sci Forschen Journals are Open Access